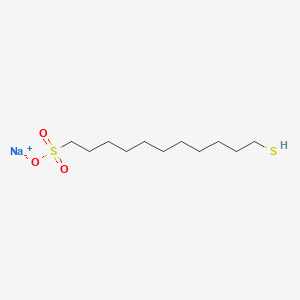
Sodium 11-mercaptoundecane-1-sulfonate
- Click on QUICK INQUIRY to receive a quote from our team of experts.
- With the quality product at a COMPETITIVE price, you can focus more on your research.
Overview
Description
Sodium 11-mercaptoundecane-1-sulfonate (MUS) is a bifunctional organosulfur compound with a linear C11 alkyl chain, terminating in a sulfonate (-SO₃⁻) group and a thiol (-SH) group. Its molecular formula is C₁₁H₂₃NaO₃S₂, and it is widely employed in nanotechnology and antiviral research due to its ability to bind metallic nanoparticles (e.g., Au, Ag) via the thiol group while the sulfonate moiety mimics heparan sulfate, a critical host-cell receptor for viruses like HSV . MUS has demonstrated enhanced antiviral activity when functionalized onto nanoparticles, particularly in blocking viral entry into host cells .
Preparation Methods
Synthetic Routes and Reaction Conditions: The synthesis of sodium 11-mercaptoundecane-1-sulfonate typically involves the reaction of 11-bromoundecane with sodium sulfite and sodium thiolate under controlled conditions . The reaction proceeds through a nucleophilic substitution mechanism, where the bromine atom is replaced by the sulfonate and mercapto groups.
Industrial Production Methods: In industrial settings, the production of this compound often involves large-scale batch reactions. The process includes the purification of the final product through recrystallization or distillation to ensure high purity and yield .
Types of Reactions:
Oxidation: this compound can undergo oxidation reactions, where the mercapto group is oxidized to form disulfides or sulfonic acids.
Reduction: The compound can be reduced to form thiols, which are useful intermediates in various chemical processes.
Substitution: It can participate in nucleophilic substitution reactions, where the sulfonate group can be replaced by other nucleophiles.
Common Reagents and Conditions:
Oxidizing Agents: Hydrogen peroxide, potassium permanganate.
Reducing Agents: Sodium borohydride, lithium aluminum hydride.
Solvents: Water, ethanol, and other polar solvents.
Major Products Formed:
Disulfides: Formed through oxidation of the mercapto group.
Thiols: Formed through reduction reactions.
Substituted Sulfonates: Formed through nucleophilic substitution reactions.
Scientific Research Applications
Antiviral Applications
Sodium 11-mercaptoundecane-1-sulfonate has been extensively studied for its antiviral properties, particularly against viruses that utilize heparan sulfate proteoglycans (HSPGs) for cell entry.
Case Study: Antiviral Activity Against Herpes Simplex Virus
A study demonstrated that gold nanoparticles coated with this compound exhibited significant antiviral activity against herpes simplex virus types 1 and 2 (HSV-1 and HSV-2). The nanoparticles were shown to block viral entry into host cells, reducing infection rates by approximately 50% in vitro. The effectiveness was attributed to the long aliphatic chain of the sulfonate ligand, which enhanced the interaction with the viral envelope .
Virus Type | Inhibition Rate | Mechanism of Action |
---|---|---|
HSV-1 | ~50% | Blockade of viral entry |
HSV-2 | ~50% | Blockade of viral entry |
Influenza A | Significant | Virucidal action through irreversible binding |
Nanoparticle Functionalization
In nanotechnology, this compound is utilized for functionalizing nanoparticles to enhance their stability and biocompatibility.
Case Study: Gold Nanoparticles in Cancer Therapy
Research has shown that gold nanoparticles functionalized with a mixture of this compound and other ligands can effectively deliver immunostimulatory agents to tumor sites. The amphiphilic nature of the ligand shell allows for the encapsulation of hydrophobic drugs without altering their chemical structure. This method significantly improves drug delivery efficiency by targeting lymph nodes draining tumors, which are crucial for initiating an immune response against cancer .
Nanoparticle Type | Ligands Used | Application |
---|---|---|
Gold Nanoparticles | 1-Octanethiol + Sodium Sulfonate | Cancer immunotherapy delivery |
Silver Nanoparticles | Sodium Sulfonate | Antiviral applications |
Biocompatibility and Low Toxicity
This compound has been noted for its low toxicity profile, making it suitable for biomedical applications. Its biocompatibility is essential when used in drug delivery systems or as a coating material for medical devices.
Case Study: Biocompatibility Assessment
Studies assessing the cytotoxicity of this compound-coated nanoparticles have shown minimal adverse effects on mammalian cells. This characteristic is crucial when developing therapeutics intended for human use, as it ensures that the carrier does not elicit harmful responses in biological systems .
Synthesis and Characterization
The synthesis of this compound typically involves a multi-step chemical process that ensures high purity and yield. Characterization techniques such as NMR spectroscopy and dynamic light scattering are employed to confirm the structure and assess the size distribution of functionalized nanoparticles.
Synthesis Overview
The synthesis generally follows these steps:
- Reaction of sodium sulfite with undecene derivatives.
- Purification through crystallization.
- Characterization using NMR and other spectroscopic methods.
Mechanism of Action
The mechanism of action of sodium 11-mercaptoundecane-1-sulfonate involves its interaction with various molecular targets. In antiviral applications, the compound can disrupt the viral envelope, preventing the virus from entering host cells . The sulfonate group interacts with viral surface proteins, while the mercapto group can form disulfide bonds, further inhibiting viral activity .
Comparison with Similar Compounds
Comparative Analysis with Structurally Similar Compounds
Structural and Functional Comparisons
The table below highlights key structural differences between MUS and analogous compounds:
Compound Name | Molecular Formula | Functional Groups | Alkyl Chain Length | Key Features |
---|---|---|---|---|
Sodium 11-mercaptoundecane-1-sulfonate (MUS) | C₁₁H₂₃NaO₃S₂ | Thiol (-SH), Sulfonate | C11 | Long flexible linker, high antiviral activity |
Sodium 2-mercaptoethanesulfonate (MES) | C₂H₅NaO₃S₂ | Thiol (-SH), Sulfonate | C2 | Short rigid linker, moderate antiviral activity |
Sodium 1-undecanesulfonate | C₁₁H₂₃NaO₃S | Sulfonate | C11 | No thiol; used in chromatography |
11-Mercaptoundecanoic acid (MUDA) | C₁₁H₂₂O₂S | Thiol (-SH), Carboxylate | C11 | Carboxylate enables pH-dependent binding |
Key Observations:
- Chain Length and Flexibility : MUS’s C11 chain provides greater conformational flexibility compared to MES (C2), enabling optimal spatial arrangement for binding viral glycoproteins .
- Functional Groups : The sulfonate group in MUS and MES mimics heparan sulfate, critical for antiviral activity, while MUDA’s carboxylate group offers distinct chemical reactivity for biosensor applications .
Antiviral Efficacy and Mechanisms
MUS-functionalized nanoparticles exhibit superior antiviral performance due to their multivalent binding capacity:
- Flexibility Impact: MUS’s extended linker enhances nanoparticle surface coverage and binding avidity, reducing HSV-1 spread by >80% in vitro compared to MES .
- Multivalency : Trimeric sulfonate scaffolds (e.g., B3C11Sulfonate) show lower potency than sulfate-terminated analogs, highlighting terminal group importance .
Toxicity and Biocompatibility
Toxicity studies of MUS-functionalized nanoparticles in Vero cells (MTT assay):
Compound | Maximum Non-Toxic Concentration (MNTC) | Viability (% vs. Control) |
---|---|---|
MUS-AgNPs | ≤20% non-viable cells | ≥80% |
MES-AgNPs | Data not reported | — |
- MUS-modified nanoparticles exhibit low cytotoxicity, making them suitable for therapeutic use .
Biological Activity
Sodium 11-mercaptoundecane-1-sulfonate (MUS) is an amphiphilic compound that has garnered attention for its diverse biological activities, particularly in antiviral and antimicrobial applications. This article explores the biological activity of MUS, supported by relevant research findings, data tables, and case studies.
Chemical Structure and Properties
MUS is characterized by its long hydrocarbon chain and sulfonate group, which contribute to its amphiphilic nature. This structure enables it to interact with various biological membranes and surfaces, enhancing its potential as a therapeutic agent.
Antiviral Activity
Mechanism of Action
MUS exhibits significant antiviral properties, particularly against viruses such as herpes simplex virus (HSV) types 1 and 2. The antiviral activity is primarily attributed to its ability to inhibit viral entry into host cells. Studies have shown that MUS functionalized nanoparticles can effectively block the attachment and penetration of HSV into Vero cells, leading to a reduction in viral infection rates .
Research Findings
A study conducted by Cagno et al. demonstrated that nanoparticles modified with MUS showed enhanced antiviral activity compared to other sulfonate ligands. The results indicated that MUS-modified nanoparticles reduced HSV-1 and HSV-2 infectivity by approximately 50%, regardless of the surface concentration of the ligand .
In another investigation, MUS was found to enhance the efficacy of gold nanoparticles against various viruses, including respiratory syncytial virus (RSV). The study reported that MUS-functionalized gold nanoparticles exhibited virucidal activity through a mechanism that involved binding to heparan sulfate proteoglycans (HSPG) on the host cell surface .
Antimicrobial Activity
MUS also demonstrates antimicrobial properties against Gram-positive bacteria such as Staphylococcus aureus. The compound's ability to disrupt bacterial membranes has been linked to its amphiphilic nature, allowing it to penetrate bacterial cell walls effectively .
Case Study: Antimicrobial Efficacy
A recent study assessed the minimum inhibitory concentration (MIC) of MUS against various bacterial strains. The results indicated that MUS had a MIC ranging from 32 µg/mL to 128 µg/mL for different strains of Staphylococcus aureus, showcasing its potential as an antimicrobial agent .
Table 1: Antiviral Efficacy of MUS-Functionalized Nanoparticles
Virus Type | Concentration (µg/mL) | Reduction in Infectivity (%) |
---|---|---|
HSV-1 | 10 | 50 |
HSV-2 | 10 | 50 |
RSV | 5 | 60 |
Table 2: Antimicrobial Activity of MUS
Bacterial Strain | Minimum Inhibitory Concentration (MIC) |
---|---|
Staphylococcus aureus | 32 - 128 µg/mL |
Escherichia coli | Not determined |
Pseudomonas aeruginosa | Not determined |
Q & A
Basic Research Questions
Q. What methodologies are recommended for synthesizing sodium 11-mercaptoundecane-1-sulfonate with high purity, and how can potential impurities be identified?
- Methodology : Use ion-pair chromatography (IPC) for purification, as sulfonate-containing compounds often require this technique to isolate ionic byproducts. Monitor reaction intermediates via FTIR to confirm thiol (-SH) and sulfonate (-SO₃⁻) group formation . For impurity profiling, combine HPLC with evaporative light scattering detection (ELSD) to detect non-UV-active contaminants, referencing USP guidelines for sulfonate reagent validation .
- Key Variables : Reaction pH (optimal range: 8–10 to prevent thiol oxidation) and solvent polarity (e.g., aqueous ethanol for solubility) .
Q. Which spectroscopic and chromatographic techniques are most effective for characterizing the structure and purity of this compound?
- Structural Confirmation :
- NMR : Use 1H NMR in D₂O to resolve peaks for the mercapto (-SH) group (δ 1.3–1.6 ppm) and sulfonate (δ 3.1–3.3 ppm) .
- XPS : Validate sulfur speciation (binding energy: ~163 eV for thiol, ~168 eV for sulfonate) to confirm coexistence of both functional groups .
- Purity Assessment : Pair reverse-phase HPLC with conductivity detection to quantify residual sodium ions and unreacted precursors .
Q. How does this compound function as a stabilizing agent in nanoparticle synthesis, and what experimental parameters govern its efficacy?
- Mechanism : The thiol group binds to metal surfaces (e.g., Au, Ag), while the sulfonate moiety enhances colloidal stability via electrostatic repulsion. Adjust ligand-to-metal molar ratios (e.g., 3:1 for AuNPs) to balance stability and aggregation .
- Critical Parameters : pH (affects thiol deprotonation), ionic strength (high concentrations screen sulfonate charges), and temperature (≥60°C accelerates ligand displacement) .
Advanced Research Questions
Q. How can researchers resolve contradictory data on the colloidal stability of nanoparticles functionalized with this compound in mixed electrolyte solutions?
- Systematic Approach :
Variable Isolation : Test individual ions (e.g., Cl⁻ vs. SO₄²⁻) to identify specific ion effects on stability .
Advanced Imaging : Use cryogenic electron tomography (cryo-ET) to visualize nanoparticle aggregation in situ, avoiding drying artifacts .
Model Validation : Apply Derjaguin-Landau-Verwey-Overbeek (DLVO) theory with corrections for steric stabilization from the sulfonate layer .
Q. What strategies optimize the use of sodium 11-meraptoundecane-1-sulfonate in surface functionalization for biosensing applications, particularly in competitive ligand environments?
- Competitive Adsorption Studies :
- Design experiments with competing thiols (e.g., mercaptohexanol) to quantify displacement kinetics using quartz crystal microbalance (QCM) .
- Use ToF-SIMS to map spatial distribution of ligands on surfaces .
- Signal-to-Noise Enhancement : Introduce zwitterionic co-ligands (e.g., carboxybetaine) to reduce nonspecific protein adsorption .
Q. How do solvent polarity and temperature influence the self-assembly of this compound monolayers on transition metal substrates?
- Solvent Effects : Polar aprotic solvents (e.g., DMF) slow monolayer formation but improve orderliness, as measured by electrochemical impedance spectroscopy (EIS) .
- Thermodynamic Analysis : Calculate Gibbs free energy changes (ΔG) for adsorption using temperature-dependent contact angle measurements .
Q. What analytical frameworks are recommended for distinguishing surface-bound vs. free this compound in complex biological matrices?
- Separation Techniques :
- Centrifugal Filtration : Use 10 kDa MWCO filters to isolate nanoparticle-bound ligands .
- LC-MS/MS : Quantify free ligands with a mass transition of m/z 293 → 96 (sulfonate fragment) .
Q. Data Contradiction Analysis
Properties
Molecular Formula |
C11H23NaO3S2 |
---|---|
Molecular Weight |
290.4 g/mol |
IUPAC Name |
sodium;11-sulfanylundecane-1-sulfonate |
InChI |
InChI=1S/C11H24O3S2.Na/c12-16(13,14)11-9-7-5-3-1-2-4-6-8-10-15;/h15H,1-11H2,(H,12,13,14);/q;+1/p-1 |
InChI Key |
UOSBKWQCFJUWDH-UHFFFAOYSA-M |
Canonical SMILES |
C(CCCCCS)CCCCCS(=O)(=O)[O-].[Na+] |
Origin of Product |
United States |
Disclaimer and Information on In-Vitro Research Products
Please be aware that all articles and product information presented on BenchChem are intended solely for informational purposes. The products available for purchase on BenchChem are specifically designed for in-vitro studies, which are conducted outside of living organisms. In-vitro studies, derived from the Latin term "in glass," involve experiments performed in controlled laboratory settings using cells or tissues. It is important to note that these products are not categorized as medicines or drugs, and they have not received approval from the FDA for the prevention, treatment, or cure of any medical condition, ailment, or disease. We must emphasize that any form of bodily introduction of these products into humans or animals is strictly prohibited by law. It is essential to adhere to these guidelines to ensure compliance with legal and ethical standards in research and experimentation.