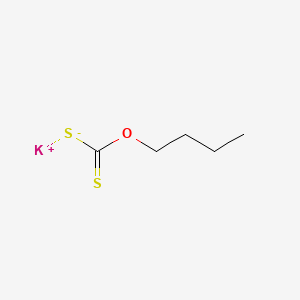
Potassium butylxanthate
Overview
Description
Potassium butylxanthate is an organosulfur compound widely used in the mining industry as a flotation agent. It is a yellowish powder with a pungent odor and is soluble in water. The compound is known for its ability to selectively bind to sulfide minerals, making it an essential reagent in the extraction of valuable metals such as copper, lead, and zinc .
Biochemical Analysis
Biochemical Properties
Potassium butylxanthate plays a significant role in biochemical reactions, particularly in the context of its interaction with enzymes, proteins, and other biomolecules. It acts as a strong nucleophile, reacting with electrophilic centers in biomolecules. This compound interacts with various enzymes, including those involved in oxidative stress responses and detoxification processes. For example, it can inhibit the activity of certain oxidoreductases by binding to their active sites, thereby affecting the redox balance within cells .
Cellular Effects
This compound has been shown to influence various types of cells and cellular processes. It can affect cell function by altering cell signaling pathways, gene expression, and cellular metabolism. In particular, this compound has been observed to induce oxidative stress in cells, leading to the activation of stress-responsive signaling pathways such as the MAPK pathway. This compound can also modulate the expression of genes involved in antioxidant defense, apoptosis, and cell cycle regulation .
Molecular Mechanism
The molecular mechanism of this compound involves its interaction with biomolecules at the molecular level. It can bind to thiol groups in proteins, leading to the formation of mixed disulfides and the inhibition of enzyme activity. This compound can also act as an electrophile, reacting with nucleophilic centers in DNA and RNA, potentially causing mutations and affecting gene expression. Additionally, this compound can modulate the activity of transcription factors, thereby influencing the transcriptional regulation of target genes .
Temporal Effects in Laboratory Settings
In laboratory settings, the effects of this compound can change over time due to its stability and degradation. This compound is relatively stable under neutral and alkaline conditions but can degrade under acidic conditions, leading to the formation of carbon disulfide and other byproducts. Long-term exposure to this compound in in vitro or in vivo studies has been associated with persistent oxidative stress and alterations in cellular function .
Preparation Methods
Synthetic Routes and Reaction Conditions
Potassium butylxanthate is synthesized by reacting potassium hydroxide with butanol to form potassium butoxide, which is then reacted with carbon disulfide to produce this compound . The reaction is typically carried out under an inert atmosphere to prevent oxidation and at a controlled temperature to ensure high yield and purity.
Industrial Production Methods
In industrial settings, the preparation of this compound involves a continuous process where potassium hydroxide and butanol are mixed in large reactors. Carbon disulfide is then added to the mixture, and the reaction is allowed to proceed under controlled conditions. The product is then purified and dried to obtain the final compound .
Chemical Reactions Analysis
Types of Reactions
Potassium butylxanthate undergoes various chemical reactions, including:
Oxidation: It can be oxidized to form dixanthogen.
Reduction: It can be reduced to form butyl alcohol and potassium carbonate.
Substitution: It can react with metal ions to form metal xanthates.
Common Reagents and Conditions
Oxidizing Agents: Potassium permanganate, hydrogen peroxide.
Reducing Agents: Sodium borohydride, lithium aluminum hydride.
Substitution Reagents: Metal salts such as copper sulfate, lead nitrate.
Major Products Formed
Oxidation: Dixanthogen.
Reduction: Butyl alcohol, potassium carbonate.
Substitution: Metal xanthates (e.g., copper xanthate, lead xanthate).
Scientific Research Applications
Potassium butylxanthate has a wide range of applications in scientific research:
Chemistry: Used as a reagent in the synthesis of various organosulfur compounds.
Biology: Studied for its potential use in biochemical assays and as a model compound for studying sulfur metabolism.
Medicine: Investigated for its potential use in drug delivery systems due to its ability to form stable complexes with metal ions.
Industry: Widely used in the mining industry as a flotation agent to enhance the extraction of valuable metals
Mechanism of Action
Potassium butylxanthate acts by selectively binding to the surface of sulfide minerals, forming a hydrophobic layer that allows the minerals to be separated from the ore during the flotation process. The compound interacts with metal ions on the mineral surface, forming stable metal-xanthate complexes that enhance the flotation efficiency .
Comparison with Similar Compounds
Similar Compounds
- Potassium ethylxanthate
- Potassium isobutylxanthate
- Potassium amylxanthate
Uniqueness
Potassium butylxanthate is unique due to its optimal balance of hydrophobicity and reactivity, making it highly effective in the flotation of a wide range of sulfide minerals. Compared to other xanthates, it offers better selectivity and higher recovery rates in mineral processing .
Properties
CAS No. |
871-58-9 |
---|---|
Molecular Formula |
C5H10KOS2 |
Molecular Weight |
189.4 g/mol |
IUPAC Name |
potassium;butoxymethanedithioate |
InChI |
InChI=1S/C5H10OS2.K/c1-2-3-4-6-5(7)8;/h2-4H2,1H3,(H,7,8); |
InChI Key |
KSVBYVVJHHRFPJ-UHFFFAOYSA-N |
SMILES |
CCCCOC(=S)[S-].[K+] |
Canonical SMILES |
CCCCOC(=S)S.[K] |
871-58-9 | |
Pictograms |
Irritant |
Related CAS |
110-50-9 (Parent) |
Origin of Product |
United States |
Synthesis routes and methods
Procedure details
Retrosynthesis Analysis
AI-Powered Synthesis Planning: Our tool employs the Template_relevance Pistachio, Template_relevance Bkms_metabolic, Template_relevance Pistachio_ringbreaker, Template_relevance Reaxys, Template_relevance Reaxys_biocatalysis model, leveraging a vast database of chemical reactions to predict feasible synthetic routes.
One-Step Synthesis Focus: Specifically designed for one-step synthesis, it provides concise and direct routes for your target compounds, streamlining the synthesis process.
Accurate Predictions: Utilizing the extensive PISTACHIO, BKMS_METABOLIC, PISTACHIO_RINGBREAKER, REAXYS, REAXYS_BIOCATALYSIS database, our tool offers high-accuracy predictions, reflecting the latest in chemical research and data.
Strategy Settings
Precursor scoring | Relevance Heuristic |
---|---|
Min. plausibility | 0.01 |
Model | Template_relevance |
Template Set | Pistachio/Bkms_metabolic/Pistachio_ringbreaker/Reaxys/Reaxys_biocatalysis |
Top-N result to add to graph | 6 |
Feasible Synthetic Routes
Disclaimer and Information on In-Vitro Research Products
Please be aware that all articles and product information presented on BenchChem are intended solely for informational purposes. The products available for purchase on BenchChem are specifically designed for in-vitro studies, which are conducted outside of living organisms. In-vitro studies, derived from the Latin term "in glass," involve experiments performed in controlled laboratory settings using cells or tissues. It is important to note that these products are not categorized as medicines or drugs, and they have not received approval from the FDA for the prevention, treatment, or cure of any medical condition, ailment, or disease. We must emphasize that any form of bodily introduction of these products into humans or animals is strictly prohibited by law. It is essential to adhere to these guidelines to ensure compliance with legal and ethical standards in research and experimentation.