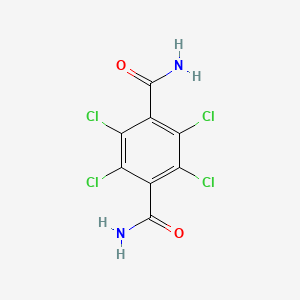
2,3,5,6-Tetrachloro-1,4-benzenedicarboxamide
Overview
Description
2,3,5,6-Tetrachloro-1,4-benzenedicarboxamide is an organic compound belonging to the family of benzamides It is characterized by the presence of four chlorine atoms and two carboxamide groups attached to a benzene ring
Preparation Methods
Synthetic Routes and Reaction Conditions
The synthesis of 2,3,5,6-Tetrachloro-1,4-benzenedicarboxamide typically involves the chlorination of 1,4-benzenedicarboxamide. One common method is the reaction of 1,4-benzenedicarboxamide with chlorine gas in the presence of a catalyst, such as iron(III) chloride, under controlled temperature and pressure conditions. The reaction proceeds as follows:
C8H6N2O2+4Cl2→C8H4Cl4N2O2+4HCl
Industrial Production Methods
In industrial settings, the production of this compound can be achieved through a continuous flow process. This involves the use of large-scale reactors where the chlorination reaction is carried out under optimized conditions to ensure high yield and purity of the final product. The process may also include purification steps such as recrystallization or distillation to remove impurities.
Chemical Reactions Analysis
Types of Reactions
2,3,5,6-Tetrachloro-1,4-benzenedicarboxamide undergoes various types of chemical reactions, including:
Substitution Reactions: The chlorine atoms in the compound can be substituted by other functional groups, such as hydroxyl or amino groups, under appropriate conditions.
Oxidation Reactions: The compound can be oxidized to form corresponding quinones or other oxidized derivatives.
Reduction Reactions: Reduction of the compound can lead to the formation of partially or fully dechlorinated products.
Common Reagents and Conditions
Substitution Reactions: Common reagents include nucleophiles such as sodium hydroxide or ammonia. The reactions are typically carried out in polar solvents like water or ethanol.
Oxidation Reactions: Oxidizing agents such as potassium permanganate or hydrogen peroxide are used. These reactions are often conducted in acidic or basic media.
Reduction Reactions: Reducing agents like sodium borohydride or lithium aluminum hydride are employed. The reactions are usually performed in anhydrous solvents like tetrahydrofuran or diethyl ether.
Major Products Formed
Substitution Reactions: Products include hydroxylated or aminated derivatives of the original compound.
Oxidation Reactions: Products include quinones or other oxidized forms of the compound.
Reduction Reactions: Products include partially or fully dechlorinated derivatives.
Scientific Research Applications
Agricultural Applications
Herbicide Development:
2,3,5,6-Tetrachloro-1,4-benzenedicarboxamide has been identified as a potential herbicide. Research indicates that its structural properties enable it to inhibit specific biochemical pathways in plants, making it effective against a range of weeds. Studies have shown that formulations containing this compound can reduce weed populations significantly without harming crop yields .
Pesticide Formulations:
In addition to herbicidal properties, this compound is being explored for use in pesticide formulations. Its ability to penetrate plant cuticles enhances the efficacy of active ingredients in pest control applications . The compound acts as a penetration enhancer, improving the delivery of pesticides through plant surfaces.
Environmental Applications
Pollution Remediation:
The compound is also being investigated for its role in the remediation of contaminated environments. Its chlorinated structure allows it to interact with various pollutants, facilitating their breakdown in soil and water. Research has demonstrated that this compound can enhance the degradation of hazardous compounds through advanced oxidation processes .
Toxicity Studies:
Given its chlorinated nature, studies on the environmental toxicity of this compound are crucial. It has been shown to exhibit acute toxicity to aquatic organisms and can bioaccumulate in the food chain. Understanding its toxicological profile helps in assessing risks associated with its use and guiding regulatory measures .
Material Science Applications
Polymer Chemistry:
In material science, this compound is utilized in the synthesis of polymers with enhanced thermal stability and chemical resistance. Its incorporation into polymer matrices can improve mechanical properties and extend the lifespan of materials used in harsh environments .
Nanocomposite Development:
The compound is also being explored for use in nanocomposite materials. When combined with nanoparticles, it can create composites with unique properties such as increased strength and reduced weight. These materials have potential applications in aerospace and automotive industries .
Case Studies
Mechanism of Action
The mechanism of action of 2,3,5,6-Tetrachloro-1,4-benzenedicarboxamide involves its interaction with specific molecular targets and pathways. The compound can bind to enzymes or receptors, leading to the modulation of their activity. For example, it may inhibit the activity of certain enzymes involved in metabolic pathways, thereby exerting its biological effects. The exact molecular targets and pathways can vary depending on the specific application and context of use.
Comparison with Similar Compounds
Similar Compounds
2,3,5,6-Tetrachloro-1,4-benzoquinone: This compound is similar in structure but contains quinone groups instead of carboxamide groups.
2,3,5,6-Tetrachloro-1,4-benzenedicarboxylic acid: This compound has carboxylic acid groups instead of carboxamide groups.
2,3,5,6-Tetrachloro-1,4-benzenedicarboxylate: This compound contains ester groups instead of carboxamide groups.
Uniqueness
2,3,5,6-Tetrachloro-1,4-benzenedicarboxamide is unique due to the presence of both chlorine atoms and carboxamide groups on the benzene ring. This combination imparts distinct chemical properties and reactivity, making it valuable for specific applications in research and industry.
Biological Activity
2,3,5,6-Tetrachloro-1,4-benzenedicarboxamide (TCBDA) is a chlorinated aromatic compound with significant implications in biological research. This compound has been studied for its potential applications in various fields, including herbicide development and biochemical research. Understanding its biological activity is crucial for assessing its safety and efficacy in practical applications.
- Molecular Formula : C8H4Cl4N2O2
- Molecular Weight : 292.93 g/mol
- CAS Number : 11324087
The biological activity of TCBDA is primarily attributed to its ability to interact with various biological targets. Its chlorinated structure allows it to participate in biochemical reactions that can disrupt normal cellular functions. The mechanism of action may involve:
- Enzyme Inhibition : TCBDA has shown potential to inhibit certain enzymes involved in metabolic processes.
- Cellular Uptake : The compound can be absorbed by cells, leading to alterations in cellular signaling pathways.
Biological Activities
Research indicates that TCBDA exhibits several biological activities:
- Antimicrobial Properties : TCBDA has been studied for its effectiveness against a range of microorganisms. Its chlorinated structure contributes to its ability to disrupt microbial cell membranes.
- Herbicidal Activity : TCBDA is utilized as a herbicide due to its ability to inhibit plant growth by interfering with photosynthesis and other metabolic pathways in plants .
- Cytotoxic Effects : Some studies have reported cytotoxic effects on various cancer cell lines, suggesting potential applications in cancer therapy. The compound's ability to induce apoptosis (programmed cell death) in these cells is of particular interest .
Case Study 1: Antimicrobial Efficacy
A study conducted by researchers evaluated the antimicrobial efficacy of TCBDA against Gram-positive and Gram-negative bacteria. The results indicated that TCBDA exhibited significant inhibitory effects on bacterial growth, particularly against Staphylococcus aureus and Escherichia coli.
Bacterial Strain | Inhibition Zone (mm) |
---|---|
Staphylococcus aureus | 15 |
Escherichia coli | 12 |
Pseudomonas aeruginosa | 10 |
Case Study 2: Herbicidal Activity
In agricultural applications, TCBDA was tested for its herbicidal properties against common weeds. The compound demonstrated effective weed control at concentrations as low as 100 ppm.
Weed Species | Control (%) at 100 ppm |
---|---|
Amaranthus retroflexus | 85 |
Chenopodium album | 78 |
Setaria viridis | 90 |
Safety and Toxicology
Despite its potential benefits, the safety profile of TCBDA must be carefully assessed. Toxicological studies have indicated that at high concentrations, TCBDA may pose risks to non-target organisms, including aquatic life. Further research is needed to establish safe usage guidelines and environmental impact assessments.
Properties
IUPAC Name |
2,3,5,6-tetrachlorobenzene-1,4-dicarboxamide | |
---|---|---|
Source | PubChem | |
URL | https://pubchem.ncbi.nlm.nih.gov | |
Description | Data deposited in or computed by PubChem | |
InChI |
InChI=1S/C8H4Cl4N2O2/c9-3-1(7(13)15)4(10)6(12)2(5(3)11)8(14)16/h(H2,13,15)(H2,14,16) | |
Source | PubChem | |
URL | https://pubchem.ncbi.nlm.nih.gov | |
Description | Data deposited in or computed by PubChem | |
InChI Key |
SSPUGLIGZZJLSW-UHFFFAOYSA-N | |
Source | PubChem | |
URL | https://pubchem.ncbi.nlm.nih.gov | |
Description | Data deposited in or computed by PubChem | |
Canonical SMILES |
C1(=C(C(=C(C(=C1Cl)Cl)C(=O)N)Cl)Cl)C(=O)N | |
Source | PubChem | |
URL | https://pubchem.ncbi.nlm.nih.gov | |
Description | Data deposited in or computed by PubChem | |
Molecular Formula |
C8H4Cl4N2O2 | |
Source | PubChem | |
URL | https://pubchem.ncbi.nlm.nih.gov | |
Description | Data deposited in or computed by PubChem | |
DSSTOX Substance ID |
DTXSID30462435 | |
Record name | 2,3,5,6-tetrachloro-1,4-benzenedicarboxamide | |
Source | EPA DSSTox | |
URL | https://comptox.epa.gov/dashboard/DTXSID30462435 | |
Description | DSSTox provides a high quality public chemistry resource for supporting improved predictive toxicology. | |
Molecular Weight |
301.9 g/mol | |
Source | PubChem | |
URL | https://pubchem.ncbi.nlm.nih.gov | |
Description | Data deposited in or computed by PubChem | |
CAS No. |
1786-85-2 | |
Record name | 2,3,5,6-tetrachloro-1,4-benzenedicarboxamide | |
Source | EPA DSSTox | |
URL | https://comptox.epa.gov/dashboard/DTXSID30462435 | |
Description | DSSTox provides a high quality public chemistry resource for supporting improved predictive toxicology. | |
Disclaimer and Information on In-Vitro Research Products
Please be aware that all articles and product information presented on BenchChem are intended solely for informational purposes. The products available for purchase on BenchChem are specifically designed for in-vitro studies, which are conducted outside of living organisms. In-vitro studies, derived from the Latin term "in glass," involve experiments performed in controlled laboratory settings using cells or tissues. It is important to note that these products are not categorized as medicines or drugs, and they have not received approval from the FDA for the prevention, treatment, or cure of any medical condition, ailment, or disease. We must emphasize that any form of bodily introduction of these products into humans or animals is strictly prohibited by law. It is essential to adhere to these guidelines to ensure compliance with legal and ethical standards in research and experimentation.