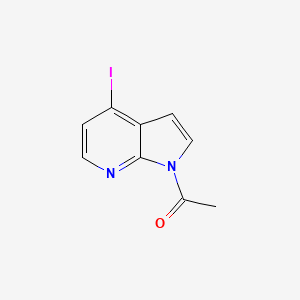
4-Iodo-1-acetyl-7-azaindole
Overview
Description
4-Iodo-1-acetyl-7-azaindole is a synthetic chemical compound with the molecular formula C9H7IN2O and a molecular weight of 286.07 g/mol. This compound belongs to the class of azaindoles, which are known for their significant biological activities and applications in various fields of research and industry . The presence of the iodine atom at the 4-position and the acetyl group at the 1-position of the azaindole ring imparts unique properties to this compound, making it a valuable tool in scientific research.
Mechanism of Action
Target of Action
The primary target of 4-Iodo-1-acetyl-7-azaindole, also known as 1-(4-iodo-1H-pyrrolo[2,3-b]pyridin-1-yl)ethanone, is the Tyrosine Protein Kinase SRC . This kinase plays a crucial role in regulating cellular processes such as proliferation, differentiation, survival, and migration .
Mode of Action
The compound interacts with its target, the Tyrosine Protein Kinase SRC, by binding to its active site . This interaction inhibits the kinase’s activity, leading to changes in the cellular processes it regulates .
Biochemical Pathways
The inhibition of Tyrosine Protein Kinase SRC affects various biochemical pathways. For instance, it can impact the MAP kinase pathway, which plays a key role in cellular proliferation . The exact downstream effects can vary depending on the specific cellular context .
Result of Action
The inhibition of Tyrosine Protein Kinase SRC by this compound can lead to antiproliferative effects, as demonstrated in studies on the MCF-7 breast cancer cell line . This suggests that the compound could potentially be used as a therapeutic agent in the treatment of certain types of cancer .
Preparation Methods
Synthetic Routes and Reaction Conditions
The synthesis of 4-Iodo-1-acetyl-7-azaindole typically involves the iodination of 1-acetyl-7-azaindole. One common method is the deprotometalation-iodolysis sequence, which uses lithium 2,2,6,6-tetramethylpiperidide as the base and the corresponding zinc diamide as an in situ trap . The reaction conditions involve the use of acetonitrile as the solvent and sodium iodide as the iodine source, with the reaction being carried out at temperatures ranging from 20°C to 85°C for 12 hours .
Industrial Production Methods
Industrial production of this compound may involve similar synthetic routes but on a larger scale. The use of microwave heating has been reported to dramatically accelerate the penultimate reaction step, an epoxide-opening-cyclization-dehydration sequence, making the process more efficient for large-scale production .
Chemical Reactions Analysis
Types of Reactions
4-Iodo-1-acetyl-7-azaindole undergoes various types of chemical reactions, including:
Substitution Reactions: The iodine atom at the 4-position can be substituted with other functional groups through nucleophilic substitution reactions.
Oxidation and Reduction Reactions: The acetyl group at the 1-position can undergo oxidation to form carboxylic acids or reduction to form alcohols.
Coupling Reactions: The compound can participate in coupling reactions, such as Suzuki coupling, to form biaryl derivatives.
Common Reagents and Conditions
Nucleophilic Substitution: Sodium iodide, acetonitrile, and lithium 2,2,6,6-tetramethylpiperidide.
Oxidation: Common oxidizing agents like potassium permanganate or chromium trioxide.
Reduction: Reducing agents such as lithium aluminum hydride or sodium borohydride.
Coupling Reactions: Palladium catalysts and boronic acids.
Major Products
Substitution Products: Various substituted azaindoles.
Oxidation Products: Carboxylic acids.
Reduction Products: Alcohols.
Coupling Products: Biaryl derivatives.
Scientific Research Applications
4-Iodo-1-acetyl-7-azaindole has a wide range of applications in scientific research, including:
Chemistry: Used as a building block for the synthesis of more complex molecules and as a reagent in various organic reactions.
Biology: Investigated for its potential as a kinase inhibitor and its role in signal transduction pathways.
Medicine: Explored for its antiproliferative and anticancer activities, particularly in the development of novel therapeutic agents.
Industry: Utilized in the production of pharmaceuticals and other fine chemicals.
Comparison with Similar Compounds
Similar Compounds
7-Azaindole: The parent compound, which lacks the iodine and acetyl groups.
4-Iodo-7-azaindole: Similar to 4-Iodo-1-acetyl-7-azaindole but without the acetyl group.
1-Acetyl-7-azaindole: Similar to this compound but without the iodine atom.
Uniqueness
This compound is unique due to the presence of both the iodine atom at the 4-position and the acetyl group at the 1-position. This combination imparts distinct chemical and biological properties, making it a valuable compound for various applications in research and industry.
Properties
IUPAC Name |
1-(4-iodopyrrolo[2,3-b]pyridin-1-yl)ethanone | |
---|---|---|
Source | PubChem | |
URL | https://pubchem.ncbi.nlm.nih.gov | |
Description | Data deposited in or computed by PubChem | |
InChI |
InChI=1S/C9H7IN2O/c1-6(13)12-5-3-7-8(10)2-4-11-9(7)12/h2-5H,1H3 | |
Source | PubChem | |
URL | https://pubchem.ncbi.nlm.nih.gov | |
Description | Data deposited in or computed by PubChem | |
InChI Key |
SDDUGTXURCFAKQ-UHFFFAOYSA-N | |
Source | PubChem | |
URL | https://pubchem.ncbi.nlm.nih.gov | |
Description | Data deposited in or computed by PubChem | |
Canonical SMILES |
CC(=O)N1C=CC2=C(C=CN=C21)I | |
Source | PubChem | |
URL | https://pubchem.ncbi.nlm.nih.gov | |
Description | Data deposited in or computed by PubChem | |
Molecular Formula |
C9H7IN2O | |
Source | PubChem | |
URL | https://pubchem.ncbi.nlm.nih.gov | |
Description | Data deposited in or computed by PubChem | |
DSSTOX Substance ID |
DTXSID80460628 | |
Record name | 1-(4-Iodo-1H-pyrrolo[2,3-b]pyridin-1-yl)ethan-1-one | |
Source | EPA DSSTox | |
URL | https://comptox.epa.gov/dashboard/DTXSID80460628 | |
Description | DSSTox provides a high quality public chemistry resource for supporting improved predictive toxicology. | |
Molecular Weight |
286.07 g/mol | |
Source | PubChem | |
URL | https://pubchem.ncbi.nlm.nih.gov | |
Description | Data deposited in or computed by PubChem | |
CAS No. |
443729-67-7 | |
Record name | 1-(4-Iodo-1H-pyrrolo[2,3-b]pyridin-1-yl)ethan-1-one | |
Source | EPA DSSTox | |
URL | https://comptox.epa.gov/dashboard/DTXSID80460628 | |
Description | DSSTox provides a high quality public chemistry resource for supporting improved predictive toxicology. | |
Record name | 4-Iodo-1-acetyl-7-azaindole | |
Source | European Chemicals Agency (ECHA) | |
URL | https://echa.europa.eu/information-on-chemicals | |
Description | The European Chemicals Agency (ECHA) is an agency of the European Union which is the driving force among regulatory authorities in implementing the EU's groundbreaking chemicals legislation for the benefit of human health and the environment as well as for innovation and competitiveness. | |
Explanation | Use of the information, documents and data from the ECHA website is subject to the terms and conditions of this Legal Notice, and subject to other binding limitations provided for under applicable law, the information, documents and data made available on the ECHA website may be reproduced, distributed and/or used, totally or in part, for non-commercial purposes provided that ECHA is acknowledged as the source: "Source: European Chemicals Agency, http://echa.europa.eu/". Such acknowledgement must be included in each copy of the material. ECHA permits and encourages organisations and individuals to create links to the ECHA website under the following cumulative conditions: Links can only be made to webpages that provide a link to the Legal Notice page. | |
Synthesis routes and methods I
Procedure details
Synthesis routes and methods II
Procedure details
Synthesis routes and methods III
Procedure details
Disclaimer and Information on In-Vitro Research Products
Please be aware that all articles and product information presented on BenchChem are intended solely for informational purposes. The products available for purchase on BenchChem are specifically designed for in-vitro studies, which are conducted outside of living organisms. In-vitro studies, derived from the Latin term "in glass," involve experiments performed in controlled laboratory settings using cells or tissues. It is important to note that these products are not categorized as medicines or drugs, and they have not received approval from the FDA for the prevention, treatment, or cure of any medical condition, ailment, or disease. We must emphasize that any form of bodily introduction of these products into humans or animals is strictly prohibited by law. It is essential to adhere to these guidelines to ensure compliance with legal and ethical standards in research and experimentation.