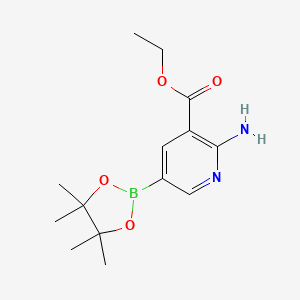
Ethyl 2-amino-5-(tetramethyl-1,3,2-dioxaborolan-2-yl)pyridine-3-carboxylate
- Click on QUICK INQUIRY to receive a quote from our team of experts.
- With the quality product at a COMPETITIVE price, you can focus more on your research.
Overview
Description
Ethyl2-amino-5-(4,4,5,5-tetramethyl-1,3,2-dioxaborolan-2-yl)nicotinate is an organic compound that features a boronate ester group. This compound is of significant interest in organic synthesis and medicinal chemistry due to its unique structural properties and reactivity.
Preparation Methods
Synthetic Routes and Reaction Conditions
The synthesis of Ethyl2-amino-5-(4,4,5,5-tetramethyl-1,3,2-dioxaborolan-2-yl)nicotinate typically involves the reaction of 2-amino-5-bromonicotinate with bis(pinacolato)diboron in the presence of a palladium catalyst. The reaction is carried out under an inert atmosphere, such as nitrogen or argon, and requires a base like potassium carbonate. The reaction mixture is usually heated to around 80-100°C for several hours to ensure complete conversion .
Industrial Production Methods
Industrial production methods for this compound are similar to laboratory synthesis but are scaled up. The use of continuous flow reactors can enhance the efficiency and yield of the reaction. Additionally, the purification process may involve crystallization or chromatography to obtain the desired purity.
Chemical Reactions Analysis
Types of Reactions
Ethyl2-amino-5-(4,4,5,5-tetramethyl-1,3,2-dioxaborolan-2-yl)nicotinate undergoes various types of chemical reactions, including:
Oxidation: The boronate ester group can be oxidized to form boronic acids.
Reduction: The nitro group can be reduced to an amine.
Substitution: The compound can participate in Suzuki-Miyaura cross-coupling reactions to form carbon-carbon bonds.
Common Reagents and Conditions
Oxidation: Hydrogen peroxide or sodium perborate in an aqueous medium.
Reduction: Palladium on carbon (Pd/C) with hydrogen gas.
Substitution: Palladium catalysts with aryl halides in the presence of a base like potassium carbonate.
Major Products
Oxidation: Boronic acids.
Reduction: Amines.
Substitution: Biaryl compounds.
Scientific Research Applications
Ethyl2-amino-5-(4,4,5,5-tetramethyl-1,3,2-dioxaborolan-2-yl)nicotinate has a wide range of applications in scientific research:
Biology: Investigated for its potential as a fluorescent probe due to the boronate ester group’s ability to interact with diols.
Industry: Utilized in the synthesis of advanced materials and polymers.
Mechanism of Action
The mechanism of action of Ethyl2-amino-5-(4,4,5,5-tetramethyl-1,3,2-dioxaborolan-2-yl)nicotinate involves its ability to form stable complexes with diols and other nucleophiles. The boronate ester group can undergo reversible reactions with diols, making it useful in sensing and drug delivery applications. The compound’s reactivity is influenced by the electronic properties of the nicotinate moiety, which can participate in various chemical transformations .
Comparison with Similar Compounds
Similar Compounds
- 4-(4,4,5,5-Tetramethyl-1,3,2-dioxaborolan-2-yl)phenol
- 1-Methyl-5-(4,4,5,5-tetramethyl-1,3,2-dioxaborolan-2-yl)-1H-imidazole
- 2-Methoxypyridine-5-boronic acid pinacol ester
Uniqueness
Ethyl2-amino-5-(4,4,5,5-tetramethyl-1,3,2-dioxaborolan-2-yl)nicotinate is unique due to its combination of a boronate ester group with a nicotinate moiety. This structural feature imparts distinct reactivity and makes it a versatile intermediate in organic synthesis. Its ability to participate in various chemical reactions and form stable complexes with diols sets it apart from other boronate esters .
Properties
Molecular Formula |
C14H21BN2O4 |
---|---|
Molecular Weight |
292.14 g/mol |
IUPAC Name |
ethyl 2-amino-5-(4,4,5,5-tetramethyl-1,3,2-dioxaborolan-2-yl)pyridine-3-carboxylate |
InChI |
InChI=1S/C14H21BN2O4/c1-6-19-12(18)10-7-9(8-17-11(10)16)15-20-13(2,3)14(4,5)21-15/h7-8H,6H2,1-5H3,(H2,16,17) |
InChI Key |
WNVKGBNFLKOMLM-UHFFFAOYSA-N |
Canonical SMILES |
B1(OC(C(O1)(C)C)(C)C)C2=CC(=C(N=C2)N)C(=O)OCC |
Origin of Product |
United States |
Disclaimer and Information on In-Vitro Research Products
Please be aware that all articles and product information presented on BenchChem are intended solely for informational purposes. The products available for purchase on BenchChem are specifically designed for in-vitro studies, which are conducted outside of living organisms. In-vitro studies, derived from the Latin term "in glass," involve experiments performed in controlled laboratory settings using cells or tissues. It is important to note that these products are not categorized as medicines or drugs, and they have not received approval from the FDA for the prevention, treatment, or cure of any medical condition, ailment, or disease. We must emphasize that any form of bodily introduction of these products into humans or animals is strictly prohibited by law. It is essential to adhere to these guidelines to ensure compliance with legal and ethical standards in research and experimentation.