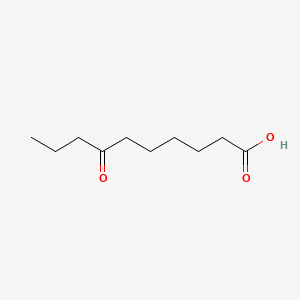
7-Oxodecanoic acid
Overview
Description
7-Oxodecanoic acid is a medium-chain fatty acid with the molecular formula C10H18O3This compound is characterized by its hydrophobic nature, making it practically insoluble in water and relatively neutral in terms of pH .
Mechanism of Action
Target of Action
7-Oxodecanoic acid is a medium-chain fatty acid Medium-chain fatty acids like this compound can cross the blood-brain barrier and be oxidized through mitochondrial β-oxidation . They have demonstrated beneficial effects on different brain diseases, such as traumatic brain injury, Alzheimer’s disease, drug-resistant epilepsy, diabetes, and cancer .
Mode of Action
It is known that medium-chain fatty acids can stimulate fatty acid synthesis . In a study with U87MG glioblastoma cells, decanoic acid (a medium-chain fatty acid) was found to influence cytosolic pathways by stimulating fatty acid synthesis .
Biochemical Pathways
This compound, as a medium-chain fatty acid, is involved in several biochemical pathways. These include the citric acid cycle, Warburg effect, glutamine/glutamate metabolism, and ketone body metabolism . The addition of medium-chain fatty acids like this compound to U87MG cells influenced these pathways .
Pharmacokinetics
Medium-chain fatty acids are known to directly enter the portal vein from the intestinal tract as free acids , suggesting that they may have good bioavailability.
Result of Action
Medium-chain fatty acids like this compound have been found to stimulate fatty acid synthesis , which could potentially influence the lipid profile of cells.
Biochemical Analysis
Biochemical Properties
7-Oxodecanoic acid plays a significant role in biochemical reactions, particularly in the oxidation of fatty acids. It is involved in the beta-oxidation pathway, where fatty acids are broken down in a stepwise fashion to produce acetyl-CoA . This process occurs in the mitochondria and involves several enzymes, including acyl-CoA dehydrogenase, enoyl-CoA hydratase, and 3-hydroxyacyl-CoA dehydrogenase . These enzymes facilitate the dehydrogenation, hydration, and further oxidation of this compound, ultimately leading to the production of energy in the form of ATP.
Cellular Effects
This compound influences various cellular processes, including cell signaling pathways, gene expression, and cellular metabolism. It has been observed to regulate the number and function of mitochondria in cells, potentially optimizing their performance . This regulation is crucial for maintaining cellular energy balance and supporting metabolic activities. Additionally, this compound’s hydrophobic nature allows it to interact with cell membranes, affecting membrane fluidity and signaling pathways.
Molecular Mechanism
At the molecular level, this compound exerts its effects through several mechanisms. It binds to specific enzymes involved in fatty acid oxidation, such as acyl-CoA dehydrogenase, and facilitates the dehydrogenation process . This binding interaction is essential for the subsequent steps in the beta-oxidation pathway. Furthermore, this compound may influence gene expression by modulating the activity of transcription factors involved in metabolic regulation . These molecular interactions highlight the compound’s role in energy production and metabolic homeostasis.
Temporal Effects in Laboratory Settings
In laboratory settings, the effects of this compound can vary over time. The compound’s stability and degradation are critical factors influencing its long-term impact on cellular function. Studies have shown that this compound is relatively stable under controlled conditions, but its degradation products may have different biochemical properties . Long-term exposure to this compound in in vitro or in vivo studies has demonstrated sustained effects on cellular metabolism and mitochondrial function . These findings underscore the importance of monitoring the temporal dynamics of this compound in experimental settings.
Dosage Effects in Animal Models
The effects of this compound in animal models vary with different dosages. At low doses, the compound may enhance mitochondrial function and support metabolic activities . At higher doses, this compound can exhibit toxic or adverse effects, potentially disrupting cellular homeostasis and causing oxidative stress . Threshold effects have been observed, indicating that there is a specific dosage range within which the compound exerts beneficial effects without causing harm. These dosage-dependent effects are crucial for understanding the compound’s therapeutic potential and safety profile.
Metabolic Pathways
This compound is involved in several metabolic pathways, including the beta-oxidation of fatty acids. This pathway involves the sequential dehydrogenation, hydration, and further oxidation of the fatty acid chain, ultimately producing acetyl-CoA . Enzymes such as acyl-CoA dehydrogenase, enoyl-CoA hydratase, and 3-hydroxyacyl-CoA dehydrogenase play key roles in these reactions . Additionally, this compound may interact with cofactors like NAD+ and FAD, which are essential for the oxidation process . These interactions highlight the compound’s role in energy metabolism and its contribution to metabolic flux.
Transport and Distribution
The transport and distribution of this compound within cells and tissues are mediated by specific transporters and binding proteins. The compound’s hydrophobic nature allows it to diffuse through cell membranes, but it may also interact with transport proteins such as carnitine acyltransferases . These proteins facilitate the transport of this compound into mitochondria, where it undergoes beta-oxidation . The distribution of this compound within tissues can influence its localization and accumulation, affecting its overall biochemical activity.
Subcellular Localization
This compound is primarily localized within the mitochondria, where it participates in the beta-oxidation of fatty acids . The compound’s targeting to mitochondria is facilitated by specific signals and transport mechanisms that direct it to this organelle. Within the mitochondria, this compound interacts with enzymes involved in fatty acid oxidation, contributing to energy production and metabolic regulation . The subcellular localization of this compound is crucial for its biochemical function and its role in maintaining cellular energy balance.
Preparation Methods
Synthetic Routes and Reaction Conditions: 7-Oxodecanoic acid can be synthesized through various methods. One common approach involves the hydrolysis of methyl 6-oxodecanoate. This process typically involves treating methyl 6-oxodecanoate with a potassium hydroxide solution at room temperature overnight . Another method involves the oxidation of γ-decalactone using potassium superoxide in the presence of tetraethylammonium bromide and dimethylformamide .
Industrial Production Methods: In industrial settings, this compound can be produced through the oxidation of decanoic acid derivatives. This process often involves the use of strong oxidizing agents such as potassium permanganate or chromium trioxide under controlled conditions to ensure high yield and purity .
Chemical Reactions Analysis
Types of Reactions: 7-Oxodecanoic acid undergoes various chemical reactions, including:
Oxidation: It can be further oxidized to form dicarboxylic acids.
Reduction: It can be reduced to form the corresponding alcohol.
Substitution: It can undergo nucleophilic substitution reactions, particularly at the carbonyl carbon.
Common Reagents and Conditions:
Oxidation: Potassium permanganate, chromium trioxide.
Reduction: Lithium aluminum hydride, sodium borohydride.
Substitution: Nucleophiles such as amines or alcohols in the presence of a base.
Major Products Formed:
Oxidation: Dicarboxylic acids.
Reduction: Alcohols.
Substitution: Amides or esters, depending on the nucleophile used.
Scientific Research Applications
7-Oxodecanoic acid has several applications in scientific research:
Comparison with Similar Compounds
- 4-Oxooctanoic acid
- 4-Oxododecanoic acid
- Decanoic acid
Comparison: 7-Oxodecanoic acid is unique due to its specific position of the keto group on the seventh carbon atom. This structural feature distinguishes it from other similar compounds such as 4-oxooctanoic acid and 4-oxododecanoic acid, which have the keto group on the fourth carbon atom. The position of the keto group can significantly influence the compound’s reactivity and its interactions with biological systems .
Properties
IUPAC Name |
7-oxodecanoic acid | |
---|---|---|
Source | PubChem | |
URL | https://pubchem.ncbi.nlm.nih.gov | |
Description | Data deposited in or computed by PubChem | |
InChI |
InChI=1S/C10H18O3/c1-2-6-9(11)7-4-3-5-8-10(12)13/h2-8H2,1H3,(H,12,13) | |
Source | PubChem | |
URL | https://pubchem.ncbi.nlm.nih.gov | |
Description | Data deposited in or computed by PubChem | |
InChI Key |
JCWIUYOZBLWKGW-UHFFFAOYSA-N | |
Source | PubChem | |
URL | https://pubchem.ncbi.nlm.nih.gov | |
Description | Data deposited in or computed by PubChem | |
Canonical SMILES |
CCCC(=O)CCCCCC(=O)O | |
Source | PubChem | |
URL | https://pubchem.ncbi.nlm.nih.gov | |
Description | Data deposited in or computed by PubChem | |
Molecular Formula |
C10H18O3 | |
Source | PubChem | |
URL | https://pubchem.ncbi.nlm.nih.gov | |
Description | Data deposited in or computed by PubChem | |
DSSTOX Substance ID |
DTXSID50956801 | |
Record name | 7-Oxodecanoic acid | |
Source | EPA DSSTox | |
URL | https://comptox.epa.gov/dashboard/DTXSID50956801 | |
Description | DSSTox provides a high quality public chemistry resource for supporting improved predictive toxicology. | |
Molecular Weight |
186.25 g/mol | |
Source | PubChem | |
URL | https://pubchem.ncbi.nlm.nih.gov | |
Description | Data deposited in or computed by PubChem | |
CAS No. |
35383-65-4 | |
Record name | 7-Oxodecanoic acid | |
Source | ChemIDplus | |
URL | https://pubchem.ncbi.nlm.nih.gov/substance/?source=chemidplus&sourceid=0035383654 | |
Description | ChemIDplus is a free, web search system that provides access to the structure and nomenclature authority files used for the identification of chemical substances cited in National Library of Medicine (NLM) databases, including the TOXNET system. | |
Record name | 7-Oxodecanoic acid | |
Source | EPA DSSTox | |
URL | https://comptox.epa.gov/dashboard/DTXSID50956801 | |
Description | DSSTox provides a high quality public chemistry resource for supporting improved predictive toxicology. | |
Retrosynthesis Analysis
AI-Powered Synthesis Planning: Our tool employs the Template_relevance Pistachio, Template_relevance Bkms_metabolic, Template_relevance Pistachio_ringbreaker, Template_relevance Reaxys, Template_relevance Reaxys_biocatalysis model, leveraging a vast database of chemical reactions to predict feasible synthetic routes.
One-Step Synthesis Focus: Specifically designed for one-step synthesis, it provides concise and direct routes for your target compounds, streamlining the synthesis process.
Accurate Predictions: Utilizing the extensive PISTACHIO, BKMS_METABOLIC, PISTACHIO_RINGBREAKER, REAXYS, REAXYS_BIOCATALYSIS database, our tool offers high-accuracy predictions, reflecting the latest in chemical research and data.
Strategy Settings
Precursor scoring | Relevance Heuristic |
---|---|
Min. plausibility | 0.01 |
Model | Template_relevance |
Template Set | Pistachio/Bkms_metabolic/Pistachio_ringbreaker/Reaxys/Reaxys_biocatalysis |
Top-N result to add to graph | 6 |
Feasible Synthetic Routes
Disclaimer and Information on In-Vitro Research Products
Please be aware that all articles and product information presented on BenchChem are intended solely for informational purposes. The products available for purchase on BenchChem are specifically designed for in-vitro studies, which are conducted outside of living organisms. In-vitro studies, derived from the Latin term "in glass," involve experiments performed in controlled laboratory settings using cells or tissues. It is important to note that these products are not categorized as medicines or drugs, and they have not received approval from the FDA for the prevention, treatment, or cure of any medical condition, ailment, or disease. We must emphasize that any form of bodily introduction of these products into humans or animals is strictly prohibited by law. It is essential to adhere to these guidelines to ensure compliance with legal and ethical standards in research and experimentation.