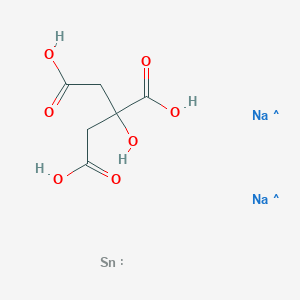
Disodium stannous citrate
- Click on QUICK INQUIRY to receive a quote from our team of experts.
- With the quality product at a COMPETITIVE price, you can focus more on your research.
Overview
Description
Disodium stannous citrate is a chemical compound with the molecular formula C6H5Na2O7Sn. It is a white crystalline solid that is highly soluble in water and has a strong reducing property. This compound is commonly used in various industrial applications, including as a reducing agent, antioxidant, and color-preserving agent .
Preparation Methods
Synthetic Routes and Reaction Conditions
Disodium stannous citrate can be synthesized through several methods. One common laboratory method involves the reaction of citric acid with stannous chloride and sodium hydroxide. The process typically involves dissolving citric acid in water, adding stannous chloride, and then neutralizing the solution with sodium hydroxide to form this compound .
Industrial Production Methods
In industrial settings, this compound is produced by reacting trisodium citrate with stannous chloride in an aqueous solution. The reaction mixture is stirred, filtered, and washed with hot water to obtain the solid product .
Chemical Reactions Analysis
Types of Reactions
Disodium stannous citrate undergoes various chemical reactions, including:
Oxidation: It is easily oxidized, especially when exposed to air.
Reduction: Acts as a reducing agent in various chemical processes.
Complexation: Forms complexes with metal ions, which is useful in metal plating and corrosion inhibition
Common Reagents and Conditions
Oxidation: Exposure to air or oxygen.
Reduction: Often used with other reducing agents or in acidic conditions.
Complexation: Reacts with metal ions in aqueous solutions
Major Products Formed
Oxidation: Forms stannic compounds.
Reduction: Reduces metal ions to their metallic state.
Complexation: Forms stable metal complexes
Scientific Research Applications
Disodium stannous citrate has a wide range of applications in scientific research:
Chemistry: Used as a reducing agent and in the synthesis of various organic compounds.
Biology: Employed in studies involving metal ion interactions and enzyme inhibition.
Medicine: Investigated for its potential antioxidant properties and its role in preserving the color and quality of food products.
Industry: Widely used in the food industry as a color-preserving agent and antioxidant, particularly in canned fruits and vegetables
Mechanism of Action
The mechanism of action of disodium stannous citrate involves its strong reducing properties. It acts by donating electrons to other molecules, thereby reducing them. This property is particularly useful in preventing oxidation and preserving the color and quality of food products. Additionally, it forms complexes with metal ions, which can inhibit enzymatic browning and microbial growth .
Comparison with Similar Compounds
Disodium stannous citrate is unique compared to other similar compounds due to its strong reducing properties and its ability to form stable complexes with metal ions. Similar compounds include:
Stannous chloride: Also a reducing agent but less stable in aqueous solutions.
Citric acid: Commonly used as an antioxidant but lacks the reducing properties of this compound.
Sodium erythorbate: Another antioxidant used in the food industry but with different chemical properties .
This compound stands out due to its combined properties of reduction, complexation, and antioxidant effects, making it highly versatile in various applications.
Properties
Molecular Formula |
C6H8Na2O7Sn |
---|---|
Molecular Weight |
356.81 g/mol |
InChI |
InChI=1S/C6H8O7.2Na.Sn/c7-3(8)1-6(13,5(11)12)2-4(9)10;;;/h13H,1-2H2,(H,7,8)(H,9,10)(H,11,12);;; |
InChI Key |
SWSJSCHGMOUPQM-UHFFFAOYSA-N |
Canonical SMILES |
C(C(=O)O)C(CC(=O)O)(C(=O)O)O.[Na].[Na].[Sn] |
Origin of Product |
United States |
Disclaimer and Information on In-Vitro Research Products
Please be aware that all articles and product information presented on BenchChem are intended solely for informational purposes. The products available for purchase on BenchChem are specifically designed for in-vitro studies, which are conducted outside of living organisms. In-vitro studies, derived from the Latin term "in glass," involve experiments performed in controlled laboratory settings using cells or tissues. It is important to note that these products are not categorized as medicines or drugs, and they have not received approval from the FDA for the prevention, treatment, or cure of any medical condition, ailment, or disease. We must emphasize that any form of bodily introduction of these products into humans or animals is strictly prohibited by law. It is essential to adhere to these guidelines to ensure compliance with legal and ethical standards in research and experimentation.