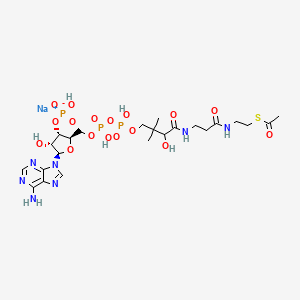
Acetylcoenzymeasodiumsalt
- Click on QUICK INQUIRY to receive a quote from our team of experts.
- With the quality product at a COMPETITIVE price, you can focus more on your research.
Overview
Description
Acetylcoenzyme A sodium salt (Acetyl-CoA, CAS 102029-73-2) is a pivotal cofactor in central metabolic pathways, serving as a carrier of acetyl groups in enzymatic reactions. It is synthesized via oxidative decarboxylation of pyruvate, β-oxidation of fatty acids, or degradation of specific amino acids . Key roles include:
- Lipid Biosynthesis: Provides carbon atoms for fatty acid synthesis and regulates pyruvate carboxylase .
- Energy Metabolism: Fuels the tricarboxylic acid (TCA) cycle in mitochondria .
- Post-Translational Modifications: Acts as a substrate for acetyltransferases, influencing histone acetylation and gene expression .
- Neurotransmitter Synthesis: Precursor to acetylcholine .
Physicochemical properties include high water solubility (100 mg/mL in H₂O) and stability at pH 3.5–5.0 under heating (100°C for 15 minutes) . Its enzymatic applications span in vitro assays, such as measuring chloramphenicol acetyltransferase (CAT) activity .
Preparation Methods
Synthetic Routes and Reaction Conditions
Acetylcoenzymeasodiumsalt is synthesized through the oxidative decarboxylation of pyruvate in mitochondria. This process involves the enzyme pyruvate dehydrogenase, which catalyzes the conversion of pyruvate to acetyl-coenzyme A. The reaction requires cofactors such as thiamine pyrophosphate, lipoic acid, and flavin adenine dinucleotide .
Industrial Production Methods
Industrial production of this compound typically involves fermentation processes using genetically engineered microorganisms. These microorganisms are designed to overproduce acetyl-coenzyme A, which is then extracted and purified for various applications .
Chemical Reactions Analysis
Types of Reactions
Acetylcoenzymeasodiumsalt undergoes several types of chemical reactions, including:
Oxidation: It can be oxidized to produce carbon dioxide and water in the Krebs cycle.
Reduction: It can be reduced to form ethanol in anaerobic conditions.
Substitution: It can participate in acetylation reactions, where the acetyl group is transferred to other molecules.
Common Reagents and Conditions
Common reagents used in reactions involving this compound include:
NAD+ (Nicotinamide adenine dinucleotide): Used in oxidation reactions.
Coenzyme A: Acts as a carrier of acyl groups in various biochemical reactions.
Major Products Formed
Major products formed from reactions involving this compound include:
Carbon dioxide and water: From oxidation in the Krebs cycle.
Ethanol: From reduction in anaerobic conditions.
Acetylated proteins: From acetylation reactions.
Scientific Research Applications
Metabolic Studies
Acetyl coenzyme A sodium salt is extensively used in metabolic research due to its role in energy production and biosynthetic pathways. Researchers utilize this compound to study:
- Fatty Acid Synthesis : Acetyl coenzyme A is a precursor for fatty acid synthesis. Studies have shown that manipulating its levels can significantly affect lipid metabolism in various cell lines, such as A549 and HepG2 .
- Energy Production : It is involved in the citric acid cycle (TCA cycle), where it contributes to ATP production. Research indicates that acetyl coenzyme A's availability can influence cell growth and energy homeostasis .
Case Study: Lipid Metabolism
A study demonstrated that depletion of acetyl coenzyme A through specific enzyme knockouts reduced palmitate synthesis, highlighting its importance in lipid metabolism .
Pharmaceutical Applications
In the pharmaceutical industry, acetyl coenzyme A sodium salt is used for:
- Drug Formulation : It enhances the bioavailability and efficacy of drugs targeting metabolic disorders. For instance, it has been incorporated into formulations for diabetes management due to its role in glucose metabolism .
- Enzyme Activity Studies : Researchers employ acetyl coenzyme A to investigate enzyme mechanisms, aiding drug discovery efforts by identifying potential drug targets .
Case Study: Metabolic Disorders
Research has indicated that acetyl coenzyme A sodium salt can improve the effectiveness of medications designed for metabolic disorders by stabilizing metabolic pathways essential for drug action.
Biochemical Research
This compound is crucial for understanding various biochemical processes:
- Enzyme Interactions : Acetyl coenzyme A serves as a substrate for numerous enzymes, facilitating studies on enzyme kinetics and regulatory mechanisms.
- Post-translational Modifications : It plays a significant role in protein acetylation, impacting gene expression and cellular signaling pathways .
Data Table: Enzyme Interactions
Enzyme Type | Role of Acetyl CoA | Reference |
---|---|---|
Histone Acetyltransferases | Modifies histones to regulate gene expression | |
Pyruvate Carboxylase | Activates conversion of pyruvate to oxaloacetate |
Food Industry Applications
Acetyl coenzyme A sodium salt is utilized as a food additive:
- Flavor Enhancement : It enhances flavors and acts as a preservative, improving product stability and shelf life.
- Nutritional Studies : Researchers study its effects on food metabolism and nutrient absorption in various dietary contexts .
Cosmetic Formulations
In cosmetics, acetyl coenzyme A sodium salt is valued for its moisturizing properties:
Mechanism of Action
Acetylcoenzymeasodiumsalt exerts its effects by acting as a carrier of acyl groups in enzymatic acetyl transfer reactions. It is formed either by the oxidative decarboxylation of pyruvate in mitochondria, by the oxidation of long-chain fatty acids, or by the oxidative degradation of certain amino acids. It is a key precursor in lipid biosynthesis and the source of all fatty acid carbons .
Comparison with Similar Compounds
Structural and Functional Analogs
Key Differences :
- Toxicity : Sodium fluoroacetate disrupts Acetyl-CoA metabolism, unlike functional analogs .
- Solubility : Long-chain acyl-CoA salts require solubilizing agents (e.g., methyl-β-cyclodextrin), whereas Acetyl-CoA sodium salt is water-soluble .
- Enzymatic Specificity : Malonyl-CoA is synthesized from Acetyl-CoA but serves a regulatory role in lipid metabolism .
Alternative Carbon Sources for Acetyl-CoA Production
Key Findings :
- Ethanol Superiority: Ethanol-derived Acetyl-CoA production in E. coli yields 34% higher PHB (polyhydroxybutyrate) than glucose/acetate due to efficient NADH generation and minimal byproducts .
- Metabolic Engineering: Optimizing adhE mutants in E. coli enhances ethanol utilization but requires chromosomal integration for stability .
Enzymatic Kinetics of Acetyl-CoA Synthases
Enzyme | Substrate (Sodium Acetate) | Km (mM) | Vmax (μM·min⁻¹·mg⁻¹) | Tissue Specificity | Reference |
---|---|---|---|---|---|
PtrACS1 | Acetate | 0.25 | 698.85 | Expressed in phloem, xylem | |
PtrACS2 | Acetate | 0.72 | 245.96 | Primarily in leaves |
Implications :
- Catalytic Efficiency : PtrACS1 has higher substrate affinity (lower Km), while PtrACS2 shows higher maximal activity .
- Peroxisomal Localization : Both enzymes contain PTS1 motifs, linking them to lipid metabolism in peroxisomes .
Engineering Acetyl-CoA Carboxylase (ACC) for Enhanced Binding
- Mutant ACCs : S343A, T347W, and S350F mutations in Streptomyces antibioticus ACC improve Acetyl-CoA binding via hydrogen bond stabilization .
- Molecular Dynamics : Mutants exhibit tighter substrate interaction (ΔG binding: -42.5 kcal/mol vs. wild-type -38.2 kcal/mol), enhancing malonyl-CoA synthesis .
Biological Activity
Acetylcoenzyme A sodium salt (Acetyl-CoA) is a pivotal metabolite in various biochemical pathways, playing a crucial role in energy metabolism and biosynthetic processes. This article delves into the biological activities of Acetyl-CoA, highlighting its mechanisms, applications, and relevant research findings.
Overview of Acetyl-CoA
Acetyl-CoA is a central metabolic intermediate formed from the oxidative decarboxylation of pyruvate, fatty acid oxidation, and the degradation of certain amino acids. It serves as an acyl donor in numerous enzymatic reactions, particularly in the citric acid cycle (TCA cycle), lipid biosynthesis, and post-translational modifications such as histone acetylation .
Mechanisms of Biological Activity
1. Energy Metabolism:
- Acetyl-CoA is essential for the TCA cycle, where it contributes to ATP production through oxidative phosphorylation. It acts as a substrate for various enzymes, including citrate synthase and pyruvate dehydrogenase .
2. Lipid Biosynthesis:
- It is a key precursor for fatty acid synthesis. Acetyl-CoA is converted to malonyl-CoA by acetyl-CoA carboxylase, initiating the fatty acid synthesis pathway .
3. Post-Translational Modifications:
- Acetyl-CoA donates acetyl groups for lysine acetylation, influencing gene expression and protein function through histone modification . This process is vital for regulating chromatin structure and transcriptional activity.
Case Studies
-
Role in Neurodegenerative Diseases:
A study investigated the role of Acetyl-CoA in cholinergic neurons during neurodegenerative conditions. The findings suggested that Acetyl-CoA levels are critical for neuronal survival, with implications for diseases such as Alzheimer's . -
Metabolic Regulation:
Research on lysine acetylation revealed that Acetyl-CoA regulates the activity of various metabolic enzymes through acetylation, impacting metabolic flux in cells under different physiological conditions .
Experimental Data
Study | Focus | Findings |
---|---|---|
Szutowicz et al. (2013) | Neurodegeneration | Acetyl-CoA levels correlate with neuronal health; low levels linked to cell death in cholinergic neurons. |
Takahashi et al. (2006) | Histone Acetylation | Nucleocytosolic acetyl-CoA synthetase is essential for histone acetylation and global transcription regulation. |
Abo Alrob & Lopaschuk (2014) | Cardiac Metabolism | Acetyl-CoA regulates fatty acid and glucose oxidation in cardiac tissues, influencing energy homeostasis. |
Assays for Biological Activity
Various assays have been developed to evaluate the biological activity of Acetyl-CoA:
- Enzyme Activity Assays: Measure the inhibition or activation of enzymes involved in metabolic pathways.
- Cell Viability Assays: Assess the impact of Acetyl-CoA on cell survival under stress conditions.
- Histone Modification Assays: Evaluate the effects of Acetyl-CoA on histone acetylation levels using Western blotting techniques.
Q & A
Basic Research Questions
Q. What are the critical considerations for preparing and storing Acetyl-CoA sodium salt stock solutions to ensure stability in enzymatic assays?
Acetyl-CoA sodium salt is highly sensitive to pH, temperature, and ionic strength. Stock solutions should be prepared in neutral buffers (e.g., phosphate or MOPS buffer) to avoid hydrolysis . Aliquot and store at –80°C to minimize freeze-thaw cycles, as repeated thawing degrades the thioester bond critical for its function. Purity (≥93% by TLC) should be verified via UV-Vis spectroscopy (λmax = 259 nm for adenine moiety) before use .
Q. How is Acetyl-CoA sodium salt utilized as a substrate in assays measuring acetyltransferase activity?
In histone acetyltransferase (HAT) assays, Acetyl-CoA serves as the acetyl donor. The reaction is typically monitored via radiometric methods (using <sup>3</sup>H- or <sup>14</sup>C-labeled Acetyl-CoA) or spectrophotometrically by coupling with Ellman’s reagent to detect free CoA-SH . Kinetic parameters (e.g., Vmax and Km) should be calculated under saturating substrate conditions to avoid underestimating enzyme efficiency .
Advanced Research Questions
Q. How can researchers resolve contradictions in reported Km values for Acetyl-CoA-dependent enzymes across studies?
Discrepancies often arise from differences in buffer composition (e.g., Tris vs. phosphate buffers alter ionic strength) or assay temperature. For example, PtrACS1 and PtrACS2 exhibit Km values of 0.25 mM and 0.72 mM, respectively, due to structural variations in their active sites . Standardize assays using validated protocols (e.g., ISO 17025) and include positive controls (e.g., purified ACS from E. coli) to ensure reproducibility .
Q. What experimental strategies are recommended for tracking Acetyl-CoA flux in metabolic pathways like the TCA cycle or fatty acid synthesis?
Isotopic tracing with <sup>13</sup>C-labeled sodium acetate (precursor to Acetyl-CoA) combined with LC-MS/MS allows quantification of Acetyl-CoA pools in specific cellular compartments . For dynamic studies, use pulse-chase experiments in perfused rat liver models to monitor incorporation into citrate or palmitate .
Q. How can researchers optimize in vitro reconstitution of Acetyl-CoA-dependent pathways (e.g., lysine acetylation) under physiologically relevant conditions?
Maintain a molar ratio of Acetyl-CoA to target proteins (e.g., histones) ≥10:1 to account for competing hydrolysis. Use ATP-regenerating systems (e.g., creatine phosphate/creatine kinase) to sustain enzymatic activity in prolonged assays . For mitochondrial studies, simulate matrix conditions with 150 mM KCl and pH 8.0 to mimic in vivo ion gradients .
Q. Data Analysis and Interpretation
Q. What statistical approaches are appropriate for analyzing dose-response relationships in Acetyl-CoA-dependent enzyme inhibition studies?
Fit data to a sigmoidal dose-response model (e.g., Hill equation) using nonlinear regression tools (GraphPad Prism, R). Account for substrate depletion by applying the Morrison equation for tight-binding inhibitors . Validate models with bootstrap resampling to assess confidence intervals for IC50 values .
Q. How should researchers address batch-to-batch variability in commercial Acetyl-CoA sodium salt when interpreting kinetic data?
Include a quality control step using enzymatic assays (e.g., citrate synthase coupled to DTNB) to verify specific activity. Normalize data to internal standards (e.g., spiked <sup>13</sup>C-Acetyl-CoA) in MS-based workflows . Report lot numbers and purity certificates to enhance cross-study comparability .
Q. Experimental Design
Q. What controls are essential for experiments investigating Acetyl-CoA’s role in protein acetylation?
- Negative controls: Omit Acetyl-CoA or use enzymatically inactive mutants (e.g., HAT catalytic site mutants).
- Specificity controls: Include non-acetylable substrate variants (e.g., lysine-to-arginine mutations).
- Interference controls: Test for thioesterase activity by incubating Acetyl-CoA with assay buffer alone .
Q. How can researchers design cofactor regeneration systems for sustained Acetyl-CoA supply in multi-enzyme cascades?
Couple with phosphotransacetylase (PTA) to regenerate Acetyl-CoA from acetate and ATP. For NADPH-dependent systems, integrate glucose-6-phosphate dehydrogenase to maintain redox balance . Monitor cofactor stability via <sup>31</sup>P-NMR to detect degradation products .
Properties
Molecular Formula |
C23H37N7NaO17P3S |
---|---|
Molecular Weight |
831.6 g/mol |
IUPAC Name |
sodium;[(2R,3S,4R,5R)-2-[[[[4-[[3-(2-acetylsulfanylethylamino)-3-oxopropyl]amino]-3-hydroxy-2,2-dimethyl-4-oxobutoxy]-hydroxyphosphoryl]oxy-hydroxyphosphoryl]oxymethyl]-5-(6-aminopurin-9-yl)-4-hydroxyoxolan-3-yl] hydrogen phosphate |
InChI |
InChI=1S/C23H38N7O17P3S.Na/c1-12(31)51-7-6-25-14(32)4-5-26-21(35)18(34)23(2,3)9-44-50(41,42)47-49(39,40)43-8-13-17(46-48(36,37)38)16(33)22(45-13)30-11-29-15-19(24)27-10-28-20(15)30;/h10-11,13,16-18,22,33-34H,4-9H2,1-3H3,(H,25,32)(H,26,35)(H,39,40)(H,41,42)(H2,24,27,28)(H2,36,37,38);/q;+1/p-1/t13-,16-,17-,18?,22-;/m1./s1 |
InChI Key |
HNLIOWFIXSPFEC-WLYMNMRISA-M |
Isomeric SMILES |
CC(=O)SCCNC(=O)CCNC(=O)C(C(C)(C)COP(=O)(O)OP(=O)(O)OC[C@@H]1[C@H]([C@H]([C@@H](O1)N2C=NC3=C(N=CN=C32)N)O)OP(=O)(O)[O-])O.[Na+] |
Canonical SMILES |
CC(=O)SCCNC(=O)CCNC(=O)C(C(C)(C)COP(=O)(O)OP(=O)(O)OCC1C(C(C(O1)N2C=NC3=C(N=CN=C32)N)O)OP(=O)(O)[O-])O.[Na+] |
Origin of Product |
United States |
Disclaimer and Information on In-Vitro Research Products
Please be aware that all articles and product information presented on BenchChem are intended solely for informational purposes. The products available for purchase on BenchChem are specifically designed for in-vitro studies, which are conducted outside of living organisms. In-vitro studies, derived from the Latin term "in glass," involve experiments performed in controlled laboratory settings using cells or tissues. It is important to note that these products are not categorized as medicines or drugs, and they have not received approval from the FDA for the prevention, treatment, or cure of any medical condition, ailment, or disease. We must emphasize that any form of bodily introduction of these products into humans or animals is strictly prohibited by law. It is essential to adhere to these guidelines to ensure compliance with legal and ethical standards in research and experimentation.