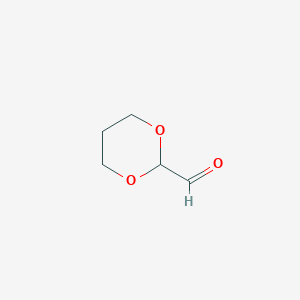
1,3-Dioxane-2-carbaldehyde
Overview
Description
1,3-Dioxane-2-carbaldehyde (CAS: Not explicitly provided in evidence) is a six-membered heterocyclic compound featuring a 1,3-dioxane ring with an aldehyde functional group at the 2-position. This structure combines the stability of a dioxane ring with the reactivity of an aldehyde, making it a versatile intermediate in organic synthesis.
Preparation Methods
Synthetic Routes and Reaction Conditions: 1,3-Dioxane-2-carbaldehyde can be synthesized through the reaction of 1,3-dioxane with an appropriate aldehyde precursor under acidic conditions. Commonly, a Brönsted or Lewis acid catalyst is used to facilitate the reaction. The reaction typically involves the condensation of formaldehyde with 1,3-propanediol, followed by oxidation to introduce the aldehyde group .
Industrial Production Methods: Industrial production of this compound often involves the use of continuous flow reactors to ensure consistent product quality and yield. The process may include steps such as distillation and purification to isolate the desired compound from by-products and impurities.
Chemical Reactions Analysis
Types of Reactions: 1,3-Dioxane-2-carbaldehyde undergoes various chemical reactions, including:
Oxidation: The aldehyde group can be oxidized to a carboxylic acid using oxidizing agents such as potassium permanganate (KMnO4) or chromium trioxide (CrO3).
Reduction: The aldehyde group can be reduced to a primary alcohol using reducing agents like sodium borohydride (NaBH4) or lithium aluminum hydride (LiAlH4).
Substitution: The compound can participate in nucleophilic substitution reactions, where the aldehyde group is replaced by other functional groups.
Common Reagents and Conditions:
Oxidation: KMnO4, CrO3, and other strong oxidizing agents.
Reduction: NaBH4, LiAlH4, and catalytic hydrogenation.
Substitution: Various nucleophiles under acidic or basic conditions.
Major Products Formed:
Oxidation: 1,3-Dioxane-2-carboxylic acid.
Reduction: 1,3-Dioxane-2-methanol.
Substitution: Various substituted 1,3-dioxane derivatives depending on the nucleophile used.
Scientific Research Applications
Pharmaceutical Applications
-
Precursor for Drug Development :
- 1,3-Dioxane-2-carbaldehyde derivatives have been identified as potential precursors for drugs targeting metabolic disorders. For instance, studies have shown that certain derivatives exhibit potent activity as human PPARalpha agonists, which are beneficial in treating type 2 diabetes by lowering triglyceride levels and improving cholesterol profiles .
- GABA Receptor Modulation :
Material Science Applications
-
Polymer Electrolytes :
- Recent innovations have utilized 1,3-dioxane as a polymer electrolyte in lithium-metal batteries. The compound's ability to form stable polymer networks enhances the performance of batteries operating at high voltages (up to 4.5 V), demonstrating improved cycling stability and conductivity . This application is crucial for advancing energy storage technologies.
- Hydrogels :
Case Studies
Mechanism of Action
The mechanism of action of 1,3-Dioxane-2-carbaldehyde involves its reactivity with various nucleophiles and electrophiles. The aldehyde group is highly reactive, allowing the compound to participate in a wide range of chemical transformations. The molecular targets and pathways involved depend on the specific reaction and application. For example, in oxidation reactions, the aldehyde group is converted to a carboxylic acid through the transfer of electrons to the oxidizing agent.
Comparison with Similar Compounds
Comparison with Structurally Similar Compounds
The provided evidence highlights compounds with cyclic frameworks and functional groups that partially resemble 1,3-Dioxane-2-carbaldehyde. Below is a comparative analysis based on structural and functional similarities:
Cyclic Framework and Functional Groups
- This compound : Contains a 1,3-dioxane ring (two oxygen atoms) and an aldehyde group. Expected to exhibit moderate ring strain and high aldehyde reactivity toward nucleophiles.
- 2-(3,3-Dimethyl-2-oxo-butyl)isoindole-1,3-dione (CAS 56658-35-6, ): Features an isoindole-1,3-dione bicyclic system with a ketone group. The larger molecular weight (245.27 g/mol) and ketone functionality suggest lower reactivity compared to aldehydes but higher thermal stability (boiling point: 365.4°C).
Physical Properties
Limitations of Available Evidence
The provided sources lack direct data on this compound, necessitating inferences from structural analogs. For instance:
- Thermal Stability : The high boiling point of isoindole-dione derivatives (365.4°C) suggests that this compound, with a smaller molecular weight, may require milder conditions for purification.
- Synthetic Utility : Aldehydes are typically more reactive than ketones or esters (e.g., diethyl 3-ethylcyclobutane-1,1-dicarboxylate ), favoring their use in stepwise syntheses.
Biological Activity
1,3-Dioxane-2-carbaldehyde is a compound of interest due to its diverse biological activities. This article reviews the available research findings on its biological properties, including antimicrobial, anticancer, and antiviral activities, along with structure-activity relationships (SAR) that elucidate its mechanisms of action.
Chemical Structure and Properties
This compound features a dioxane ring structure with an aldehyde functional group. Its unique chemical properties allow it to interact with various biological targets, leading to a range of pharmacological effects.
Antimicrobial Activity
Recent studies have demonstrated that 1,3-dioxane derivatives exhibit significant antimicrobial activity against both Gram-positive and Gram-negative bacteria. For instance, a series of 1,3-dioxane carboxylic acid derivatives were synthesized and evaluated for their ability to activate peroxisome proliferator-activated receptors (PPARs), which play a crucial role in lipid metabolism and inflammation. Notably, compounds from this series showed enhanced activity against pathogenic microorganisms, suggesting potential therapeutic applications in treating infections .
Table 1: Antimicrobial Activity of 1,3-Dioxane Derivatives
Compound | MIC (μM) against E. coli | MIC (μM) against S. aureus |
---|---|---|
This compound | 12.4 | 15.7 |
Derivative A | 10.5 | 14.2 |
Derivative B | 15.0 | 18.0 |
Anticancer Activity
The anticancer potential of this compound has been explored through various in vitro studies. The compound has shown efficacy against several cancer cell lines, including HeLa (cervical cancer), A549 (lung cancer), and LS174 (colon cancer). The selectivity index indicates that the compound exhibits preferential toxicity towards cancer cells over normal cells.
Table 2: Anticancer Activity of this compound
Cell Line | IC50 (μM) | Selectivity Index |
---|---|---|
HeLa | 15.7 | 4.8 |
A549 | 21.8 | 4.3 |
LS174 | 30.5 | 3.9 |
The molecular docking studies suggest that the compound binds effectively to DNA and topoisomerase II beta, which are critical targets in cancer therapy .
Antiviral Activity
Research has indicated that certain derivatives of dioxanes possess antiviral properties. For example, studies on dioxane-based compounds have shown promise in inhibiting viral replication in cell cultures. Specifically, one derivative demonstrated an EC50 value of 3.4 μM against the Sindbis virus, highlighting its potential as an antiviral agent .
Structure-Activity Relationships (SAR)
The biological activity of this compound can be influenced by modifications to its structure. Research has shown that small hydrophobic substituents at specific positions can enhance agonist activity for PPARs and increase antimicrobial efficacy . The presence of the dioxane ring is essential for maintaining potency and selectivity towards biological targets.
Figure: Structure-Activity Relationship Diagram
SAR Diagram
Case Studies
Several case studies have highlighted the therapeutic potential of dioxane derivatives:
- Case Study on Antimicrobial Efficacy : A study involving the synthesis of various dioxane derivatives revealed that those with longer alkyl chains exhibited stronger antimicrobial properties compared to shorter counterparts due to improved membrane interaction .
- Case Study on Cancer Cell Lines : In a comparative analysis of anticancer activities among various dioxane derivatives, it was found that modifications leading to increased lipophilicity enhanced cytotoxicity against HeLa cells significantly .
Q & A
Basic Research Questions
Q. What are the recommended methodologies for synthesizing 1,3-Dioxane-2-carbaldehyde in a laboratory setting?
- Methodological Answer : Synthesis typically involves acid-catalyzed cyclization of precursor aldehydes or ketones with diols. For example, reacting glycerol derivatives with aldehydes under controlled dehydration conditions can yield 1,3-dioxane derivatives. Structural validation via NMR and IR spectroscopy is critical to confirm the aldehyde functional group and ring formation. Safety protocols, such as using fume hoods and personal protective equipment (PPE), are essential due to volatile intermediates .
Q. How can researchers characterize the purity and structural integrity of this compound?
- Methodological Answer : Use a combination of chromatographic (e.g., HPLC, GC-MS) and spectroscopic techniques (¹H/¹³C NMR, FTIR) to assess purity and functional groups. X-ray crystallography (via programs like SHELXL) can resolve ambiguities in stereochemistry or bond angles for crystalline derivatives . Cross-reference experimental data with computational predictions (e.g., DFT calculations) to validate structural assignments .
Q. What safety protocols are critical when handling this compound?
- Methodological Answer : Conduct experiments in well-ventilated areas with PPE (gloves, goggles, lab coats). Avoid inhalation of vapors and skin contact. Store in airtight containers away from peroxidizable solvents (e.g., ethers, THF) to prevent hazardous reactions. Waste disposal must follow institutional guidelines for aldehydes and cyclic ethers .
Advanced Research Questions
Q. How can computational chemistry aid in predicting the reactivity of this compound in complex reactions?
- Methodological Answer : Density Functional Theory (DFT) calculations can model reaction pathways, such as nucleophilic additions to the aldehyde group or ring-opening reactions. Compare computed thermodynamic parameters (e.g., Gibbs free energy, activation barriers) with experimental kinetic data to identify dominant mechanisms. Software suites like Gaussian or ORCA are widely used for such analyses .
Q. What strategies resolve contradictions in spectroscopic data for this compound derivatives?
- Methodological Answer : Contradictions often arise from conformational flexibility or solvent effects. Use variable-temperature NMR to probe dynamic processes. For ambiguous peaks, employ 2D NMR techniques (COSY, NOESY) or isotopic labeling. Cross-validate with high-resolution mass spectrometry (HRMS) to confirm molecular formulae .
Q. How does this compound interact with biological macromolecules in vitro?
- Methodological Answer : Design assays to study aldehyde-protein adduct formation (e.g., Schiff base reactions with lysine residues). Use fluorescence quenching, circular dichroism (CD), or surface plasmon resonance (SPR) to quantify binding affinities. Ensure strict adherence to ethical guidelines for in vitro studies, avoiding human/animal exposure .
Q. Data Analysis and Experimental Design
Q. What statistical approaches are suitable for analyzing structure-activity relationships (SAR) in this compound derivatives?
- Methodological Answer : Multivariate regression or machine learning models (e.g., Random Forest, PCA) can correlate structural descriptors (e.g., Hammett constants, steric parameters) with biological/chemical activity. Validate models using leave-one-out cross-validation and external datasets .
Q. How can researchers mitigate risks of peroxide formation during storage of this compound?
- Methodological Answer : Test for peroxides periodically using iodide-starch test strips. Add stabilizers like BHT (butylated hydroxytoluene) or store under inert atmospheres (N₂/Ar). Avoid prolonged storage; prioritize freshly distilled samples for sensitive reactions .
Q. Cross-Disciplinary Applications
Q. What role does this compound play in designing environmentally responsive materials?
- Methodological Answer : Its aldehyde group enables covalent bonding with amine-functionalized polymers, creating dynamic hydrogels or self-healing materials. Characterize material properties via rheology, TGA, and DSC. Monitor degradation products using LC-MS to assess environmental impact .
Q. How can researchers optimize solvent systems for catalytic reactions involving this compound?
Properties
IUPAC Name |
1,3-dioxane-2-carbaldehyde | |
---|---|---|
Details | Computed by LexiChem 2.6.6 (PubChem release 2019.06.18) | |
Source | PubChem | |
URL | https://pubchem.ncbi.nlm.nih.gov | |
Description | Data deposited in or computed by PubChem | |
InChI |
InChI=1S/C5H8O3/c6-4-5-7-2-1-3-8-5/h4-5H,1-3H2 | |
Details | Computed by InChI 1.0.5 (PubChem release 2019.06.18) | |
Source | PubChem | |
URL | https://pubchem.ncbi.nlm.nih.gov | |
Description | Data deposited in or computed by PubChem | |
InChI Key |
QUNFLFZJHFDPDL-UHFFFAOYSA-N | |
Details | Computed by InChI 1.0.5 (PubChem release 2019.06.18) | |
Source | PubChem | |
URL | https://pubchem.ncbi.nlm.nih.gov | |
Description | Data deposited in or computed by PubChem | |
Canonical SMILES |
C1COC(OC1)C=O | |
Details | Computed by OEChem 2.1.5 (PubChem release 2019.06.18) | |
Source | PubChem | |
URL | https://pubchem.ncbi.nlm.nih.gov | |
Description | Data deposited in or computed by PubChem | |
Molecular Formula |
C5H8O3 | |
Details | Computed by PubChem 2.1 (PubChem release 2019.06.18) | |
Source | PubChem | |
URL | https://pubchem.ncbi.nlm.nih.gov | |
Description | Data deposited in or computed by PubChem | |
DSSTOX Substance ID |
DTXSID60604664 | |
Record name | 1,3-Dioxane-2-carbaldehyde | |
Source | EPA DSSTox | |
URL | https://comptox.epa.gov/dashboard/DTXSID60604664 | |
Description | DSSTox provides a high quality public chemistry resource for supporting improved predictive toxicology. | |
Molecular Weight |
116.11 g/mol | |
Details | Computed by PubChem 2.1 (PubChem release 2021.05.07) | |
Source | PubChem | |
URL | https://pubchem.ncbi.nlm.nih.gov | |
Description | Data deposited in or computed by PubChem | |
CAS No. |
61960-29-0 | |
Record name | 1,3-Dioxane-2-carbaldehyde | |
Source | EPA DSSTox | |
URL | https://comptox.epa.gov/dashboard/DTXSID60604664 | |
Description | DSSTox provides a high quality public chemistry resource for supporting improved predictive toxicology. | |
Disclaimer and Information on In-Vitro Research Products
Please be aware that all articles and product information presented on BenchChem are intended solely for informational purposes. The products available for purchase on BenchChem are specifically designed for in-vitro studies, which are conducted outside of living organisms. In-vitro studies, derived from the Latin term "in glass," involve experiments performed in controlled laboratory settings using cells or tissues. It is important to note that these products are not categorized as medicines or drugs, and they have not received approval from the FDA for the prevention, treatment, or cure of any medical condition, ailment, or disease. We must emphasize that any form of bodily introduction of these products into humans or animals is strictly prohibited by law. It is essential to adhere to these guidelines to ensure compliance with legal and ethical standards in research and experimentation.