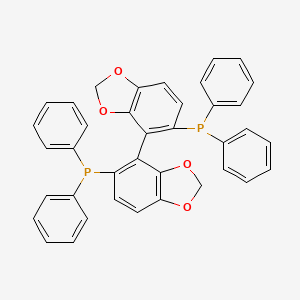
Segphos
Overview
Description
Segphos, a chiral bisphosphine ligand developed by Takasago International Corporation, is widely used in asymmetric catalysis due to its unique structural and electronic properties. Its core consists of a biphenyl backbone integrated with 1,3-benzodioxole rings, which enforce a narrow dihedral angle (64.99° in Ru complexes) between the aromatic planes . This configuration creates a rigid, C2-symmetric chiral environment that enhances stereochemical control in catalytic reactions. This compound derivatives, such as DM-Segphos (3,5-dimethylphenyl substituents) and DTBM-Segphos (3,5-di-tert-butyl-4-methoxyphenyl substituents), further modulate steric and electronic effects, enabling applications in hydrogenation, cycloaddition, and C–H functionalization .
Preparation Methods
Segphos can be synthesized through several synthetic routes. One common method involves the reaction of 4,4′-bi-1,3-benzodioxole with diphenylphosphine under specific conditions to form the desired product . Industrial production methods often involve the use of transition metal catalysts to facilitate the reaction and improve yield and purity .
Chemical Reactions Analysis
Segphos undergoes various types of chemical reactions, including:
Hydrogenation: this compound is commonly used in asymmetric hydrogenation reactions.
Substitution: This compound can participate in substitution reactions where its phosphine groups are replaced with other functional groups.
Oxidation and Reduction: This compound can also undergo oxidation and reduction reactions, often in the presence of specific reagents and under controlled conditions.
Common reagents used in these reactions include hydrogen gas, transition metal catalysts, and various organic solvents. The major products formed from these reactions are often chiral compounds with high enantioselectivity .
Scientific Research Applications
Asymmetric Hydrogenation
One of the primary applications of Segphos is in asymmetric hydrogenation , where it serves as a ligand for transition metals such as ruthenium. The following table summarizes key findings related to its performance in this area:
Photonic Applications
Recent studies have explored the use of this compound in photonic materials, particularly for its properties in persistent luminescence and circularly polarized light emission. The following highlights its capabilities:
- Janus this compound : Integrates persistent photogenic radicaloids with enhanced energy transfer mechanisms, making it suitable for optical applications such as displays and information encryption .
- Photonic Radicaloids : Exhibits long-lasting phosphorescence due to efficient intersystem crossing (ISC) and triplet energy transfer, which can be harnessed for advanced photonic devices .
Case Study 1: Asymmetric Dimerization
A study demonstrated the effectiveness of this compound in the asymmetric dimerization of aryl halides. The process yielded high enantiomeric excess (ee) and showcased a broad substrate scope, indicating its potential for developing new enantioenriched biaryl bisphosphine ligands.
Case Study 2: Nickel-Catalyzed Reactions
Research focused on the synthesis of [(R)-DTBM-SEGPHOS]NiCl2 revealed its efficiency in enantioselective carbon–carbon bond-forming reactions. The catalytic system achieved significant yields while maintaining stereochemical integrity, showcasing the practical utility of this compound in complex organic syntheses .
Case Study 3: Mechanistic Insights
Investigations into copper-catalyzed hydroboration mechanisms highlighted how this compound as a ligand facilitated the formation of dimeric copper hydride complexes. These complexes were crucial for achieving enantioselectivity during reactions involving vinylarenes, further emphasizing the ligand's role in enhancing catalytic performance .
Mechanism of Action
The mechanism by which Segphos exerts its effects involves the formation of chiral metal complexes. These complexes create an asymmetric environment that facilitates the selective transformation of substrates into chiral products . The molecular targets and pathways involved in these reactions depend on the specific metal and substrate used. The unique structure of this compound allows for weakly attractive interactions between the catalyst and substrate, enhancing the overall catalytic activity and selectivity .
Comparison with Similar Compounds
Structural and Electronic Comparisons
Table 1: Key Structural Parameters of Segphos and Analogous Ligands
Ligand | Dihedral Angle (°) | Substituents on P | Key Features |
---|---|---|---|
This compound | 64.99 | Ph | Narrow dihedral angle; 1,3-benzodioxole backbone |
BINAP | 73.49 | Ph | Broader dihedral angle; binaphthyl backbone |
H8-BINAP | ~70–72 | Ph | Hydrogenated naphthyl rings; increased rigidity |
BPhPHOS-OMe | N/A | OMe | Methoxy groups; unlocked conformation |
This compound-CF2 | 79.6 | CF2 | Fluorinated bridge; disrupted radical emission |
- BINAP : this compound outperforms BINAP in enantioselectivity due to its narrower dihedral angle, which improves spatial discrimination of substrates. For example, in the hydrogenation of α-keto esters, this compound achieves 97.6% ee vs. 87.0% ee for BINAP .
- BPhPHOS-OMe: Unlike this compound, BPhPHOS-OMe lacks a locked bridge structure, leading to free rotation of methoxy groups and reduced stereochemical control.
- This compound-CF2: Fluorination of the bridge increases the dihedral angle to 79.6°, disrupting non-covalent interactions (NCIs) and eliminating photogenic radical behavior observed in this compound .
Catalytic Performance
Table 2: Enantioselectivity and Yields in Representative Reactions
- Hydrogenation : this compound-based Ru catalysts operate at lower pressures (~350 psig) compared to BINAP, with superior enantioselectivity in β-keto ester reductions (98.5% ee vs. 95.9% ee) .
- Annulation Reactions : The dihedral angle of this compound complements the TADDOL-derived scaffold in Ru catalysis, enabling CH-π interactions that enhance rigidity and enantioselectivity (89% ee vs. 31% ee for mismatched (R)-Segphos) .
- 1,3-Dipolar Cycloaddition : DTBM-Segphos’s bulky substituents improve steric shielding, achieving 96% ee at room temperature, whereas BINAP yields poor selectivity (28% ee) .
Electronic and Steric Tuning
- DM-Segphos : The 3,5-dimethylphenyl groups increase electron density at the phosphorus center, enhancing reactivity in Ni-catalyzed α-arylations of ketones .
- DTBM-Segphos : The 3,5-di-tert-butyl-4-methoxyphenyl substituents introduce steric bulk, critical for stabilizing Ir-hydride intermediates in N–H bond additions .
Biological Activity
Segphos, specifically (R)-Dtbm-segphos, is a chiral bidentate phosphine ligand that plays a significant role in asymmetric catalysis within organic synthesis. While it is primarily recognized for its catalytic properties, its implications in biological activity arise through its use in synthesizing biologically active compounds. This article explores the biological activity associated with this compound, its applications in various reactions, and relevant case studies.
Chemical Structure and Properties
Chemical Formula : C74H100O8P2
Molecular Weight : 1,179.55 g/mol
This compound features bulky tert-butyl and methoxy groups on phenyl rings, enhancing its steric properties and making it effective in catalysis. Its structure allows for effective coordination with transition metals, which is critical for its function as a ligand in various reactions.
Applications in Asymmetric Catalysis
This compound is widely utilized in several key chemical reactions that yield enantiomerically enriched products. Some notable applications include:
- Asymmetric Hydroacylation : this compound is employed as a catalyst in the asymmetric intramolecular hydroacylation of ketoaldehydes, facilitating the formation of new compounds through [3,3]-sigmatropic rearrangement processes.
- Synthesis of Dihydrobenzofurans : It is also used in synthesizing dihydrobenzofurans and dihydronaphthofurans, which are important heterocyclic compounds found in many natural products and pharmaceuticals.
- Copper-Catalyzed Reactions : Mechanistic studies have shown that this compound can form stable complexes with copper, enhancing the efficiency of reactions such as hydroboration of vinylarenes and internal alkenes .
Biological Activity Through Synthesis
While this compound itself may not exhibit direct biological activity, its role as a catalyst enables the synthesis of compounds with significant biological properties. The chiral intermediates produced through its use are essential for developing pharmaceuticals that require specific stereochemistry for efficacy and safety.
Table 1: Summary of Biological Applications
Reaction Type | Product Type | Biological Relevance |
---|---|---|
Asymmetric Hydroacylation | Chiral ketones | Potential therapeutic agents |
Dihydrobenzofuran Synthesis | Heterocyclic compounds | Found in natural products and pharmaceuticals |
Copper-Catalyzed Hydroboration | Chiral boronate esters | Important for drug development |
Case Studies
-
Asymmetric Dimerization :
Research highlighted the use of this compound in the asymmetric dimerization of aryl halides. The process yielded high enantiomeric excess (ee) and demonstrated broad scope regarding substrate variation. This method has implications for developing new enantioenriched biaryl bisphosphine ligands . -
Nickel-Catalyzed Reactions :
A study focused on the synthesis of [(R)-DTBM-SEGPHOS]NiCl2 demonstrated its effectiveness in enantioselective carbon–carbon bond-forming reactions. The catalytic system produced significant yields while retaining stereochemical integrity . -
Mechanistic Insights :
Investigations into the copper-catalyzed hydroboration mechanism revealed that with this compound as a ligand, dimeric copper hydride complexes were formed, which played a crucial role in achieving enantioselectivity during reactions involving vinylarenes .
Q & A
Basic Research Questions
Q. How is Segphos synthesized and characterized in laboratory settings?
this compound synthesis typically involves palladium-catalyzed asymmetric cross-couplings or chiral resolution techniques. Key characterization methods include:
- Nuclear Magnetic Resonance (NMR) : To confirm stereochemistry and purity (e.g., P NMR for phosphorus environments) .
- X-ray Crystallography : For definitive structural elucidation of chiral centers .
- High-Performance Liquid Chromatography (HPLC) : To assess enantiomeric excess using chiral columns . Methodological Tip: Ensure rigorous exclusion of moisture and oxygen during synthesis to prevent ligand degradation .
Q. What are the primary applications of this compound in asymmetric catalysis?
this compound is widely used in enantioselective hydrogenation and cross-coupling reactions. Key applications include:
- C–C Bond Formation : As a ligand in Suzuki-Miyaura couplings .
- Hydrogenation of Ketones : Achieving high enantiomeric excess (>95%) in pharmaceutical intermediates . Experimental Design: Optimize reaction conditions (e.g., solvent polarity, temperature) using Design of Experiments (DoE) to maximize yield and selectivity .
Q. How do researchers ensure reproducibility in this compound-based catalytic systems?
Reproducibility requires:
- Standardized Protocols : Detailed documentation of catalyst loading, substrate ratios, and reaction times .
- Control Experiments : Including blank reactions to rule out non-catalytic pathways .
- Data Sharing : Raw NMR spectra and crystallographic data should be archived in supplementary materials .
Advanced Research Questions
Q. What mechanistic insights explain this compound’ enantioselectivity in asymmetric catalysis?
Enantioselectivity arises from steric and electronic interactions between the chiral ligand and substrate. Advanced methods to probe this include:
- DFT Calculations : Modeling transition states to predict stereochemical outcomes .
- In Situ Spectroscopy : Monitoring reaction intermediates via IR or Raman spectroscopy . Data Contradiction Analysis: Discrepancies between computational and experimental results may indicate overlooked solvent effects or counterion influences .
Q. How can this compound be modified to enhance catalytic activity in challenging substrates?
Ligand optimization strategies:
- Steric Tuning : Introducing bulkier substituents (e.g., aryl vs. alkyl groups) to modulate steric hindrance .
- Electronic Modulation : Incorporating electron-withdrawing/donating groups to adjust metal-ligand bond strength . Validation: Compare turnover numbers (TON) and enantiomeric excess (ee) across modified ligands using ANOVA to identify statistically significant improvements .
Q. What are the common pitfalls in interpreting spectroscopic data for this compound-metal complexes?
Challenges include:
- Overlapping NMR Peaks : Use C-H HSQC or DOSY experiments to resolve complex spectra .
- Paramagnetic Broadening : Avoid ferromagnetic impurities in metal precursors . Methodology: Cross-validate data with multiple techniques (e.g., EPR for paramagnetic species) .
Q. How do researchers address contradictory results in this compound-mediated reactions across different studies?
Contradictions often stem from:
- Substrate Purity : Trace impurities in starting materials can alter reaction pathways .
- Atmospheric Conditions : Moisture-sensitive reactions may yield inconsistent results if not rigorously controlled . Resolution Strategy: Conduct meta-analyses of published data to identify confounding variables (e.g., solvent grade, catalyst age) .
Q. Data Analysis and Interpretation
Q. What statistical methods are recommended for analyzing enantioselectivity data in this compound studies?
- Multivariate Analysis : Principal Component Analysis (PCA) to correlate reaction parameters with ee .
- Error Propagation : Calculate confidence intervals for ee measurements using bootstrap resampling . Best Practice: Report raw data alongside processed results to enable independent validation .
Q. How can computational chemistry complement experimental studies of this compound?
- Molecular Dynamics (MD) : Simulate ligand flexibility under reaction conditions .
- Docking Studies : Predict substrate binding modes in catalytic pockets . Limitations: Validate computational models against experimental kinetics (e.g., Eyring plots) .
Q. Research Design and Literature Gaps
Q. What unresolved questions exist in the application of this compound to C–H activation reactions?
Current gaps include:
- Mechanistic Understanding : The role of directing groups in regioselectivity remains debated .
- Substrate Scope : Limited data on heteroaromatic and sterically hindered substrates .
Research Proposal: Use high-throughput screening to identify optimal ligand-substrate pairs .
Q. How can researchers design studies to bridge theoretical and practical knowledge gaps in this compound chemistry?
- Hybrid Approaches : Combine kinetic studies (e.g., rate law determination) with computational modeling .
- Collaborative Frameworks : Partner with crystallography and spectroscopy specialists to obtain multidimensional data .
Q. Tables for Quick Reference
Analytical Technique | Application to this compound | Key Considerations | Reference |
---|---|---|---|
P NMR | Ligand purity and coordination | Use deuterated solvents for clarity | |
X-ray Crystallography | Absolute configuration determination | Requires high-quality single crystals | |
HPLC with Chiral Columns | Enantiomeric excess measurement | Calibrate with racemic mixtures |
Properties
IUPAC Name |
[4-(5-diphenylphosphanyl-1,3-benzodioxol-4-yl)-1,3-benzodioxol-5-yl]-diphenylphosphane | |
---|---|---|
Source | PubChem | |
URL | https://pubchem.ncbi.nlm.nih.gov | |
Description | Data deposited in or computed by PubChem | |
InChI |
InChI=1S/C38H28O4P2/c1-5-13-27(14-6-1)43(28-15-7-2-8-16-28)33-23-21-31-37(41-25-39-31)35(33)36-34(24-22-32-38(36)42-26-40-32)44(29-17-9-3-10-18-29)30-19-11-4-12-20-30/h1-24H,25-26H2 | |
Source | PubChem | |
URL | https://pubchem.ncbi.nlm.nih.gov | |
Description | Data deposited in or computed by PubChem | |
InChI Key |
RZZDRSHFIVOQAF-UHFFFAOYSA-N | |
Source | PubChem | |
URL | https://pubchem.ncbi.nlm.nih.gov | |
Description | Data deposited in or computed by PubChem | |
Canonical SMILES |
C1OC2=C(O1)C(=C(C=C2)P(C3=CC=CC=C3)C4=CC=CC=C4)C5=C(C=CC6=C5OCO6)P(C7=CC=CC=C7)C8=CC=CC=C8 | |
Source | PubChem | |
URL | https://pubchem.ncbi.nlm.nih.gov | |
Description | Data deposited in or computed by PubChem | |
Molecular Formula |
C38H28O4P2 | |
Source | PubChem | |
URL | https://pubchem.ncbi.nlm.nih.gov | |
Description | Data deposited in or computed by PubChem | |
DSSTOX Substance ID |
DTXSID101145953 | |
Record name | (R)-(+)-SEGPHOS | |
Source | EPA DSSTox | |
URL | https://comptox.epa.gov/dashboard/DTXSID101145953 | |
Description | DSSTox provides a high quality public chemistry resource for supporting improved predictive toxicology. | |
Molecular Weight |
610.6 g/mol | |
Source | PubChem | |
URL | https://pubchem.ncbi.nlm.nih.gov | |
Description | Data deposited in or computed by PubChem | |
CAS No. |
244261-66-3, 210169-54-3 | |
Record name | 1,1′-[(4R)-[4,4′-Bi-1,3-benzodioxole]-5,5′-diyl]bis[1,1-diphenylphosphine] | |
Source | CAS Common Chemistry | |
URL | https://commonchemistry.cas.org/detail?cas_rn=244261-66-3 | |
Description | CAS Common Chemistry is an open community resource for accessing chemical information. Nearly 500,000 chemical substances from CAS REGISTRY cover areas of community interest, including common and frequently regulated chemicals, and those relevant to high school and undergraduate chemistry classes. This chemical information, curated by our expert scientists, is provided in alignment with our mission as a division of the American Chemical Society. | |
Explanation | The data from CAS Common Chemistry is provided under a CC-BY-NC 4.0 license, unless otherwise stated. | |
Record name | (R)-(+)-SEGPHOS | |
Source | EPA DSSTox | |
URL | https://comptox.epa.gov/dashboard/DTXSID101145953 | |
Description | DSSTox provides a high quality public chemistry resource for supporting improved predictive toxicology. | |
Record name | 4,4'-Bi-1,3-benzodioxole-5,5'-diylbis(diphenylphosphine) | |
Source | European Chemicals Agency (ECHA) | |
URL | https://echa.europa.eu/information-on-chemicals | |
Description | The European Chemicals Agency (ECHA) is an agency of the European Union which is the driving force among regulatory authorities in implementing the EU's groundbreaking chemicals legislation for the benefit of human health and the environment as well as for innovation and competitiveness. | |
Explanation | Use of the information, documents and data from the ECHA website is subject to the terms and conditions of this Legal Notice, and subject to other binding limitations provided for under applicable law, the information, documents and data made available on the ECHA website may be reproduced, distributed and/or used, totally or in part, for non-commercial purposes provided that ECHA is acknowledged as the source: "Source: European Chemicals Agency, http://echa.europa.eu/". Such acknowledgement must be included in each copy of the material. ECHA permits and encourages organisations and individuals to create links to the ECHA website under the following cumulative conditions: Links can only be made to webpages that provide a link to the Legal Notice page. | |
Record name | 4,4'-Bi-1,3-benzodioxole-5,5'-diylbis(diphenylphosphine) | |
Source | European Chemicals Agency (ECHA) | |
URL | https://echa.europa.eu/information-on-chemicals | |
Description | The European Chemicals Agency (ECHA) is an agency of the European Union which is the driving force among regulatory authorities in implementing the EU's groundbreaking chemicals legislation for the benefit of human health and the environment as well as for innovation and competitiveness. | |
Explanation | Use of the information, documents and data from the ECHA website is subject to the terms and conditions of this Legal Notice, and subject to other binding limitations provided for under applicable law, the information, documents and data made available on the ECHA website may be reproduced, distributed and/or used, totally or in part, for non-commercial purposes provided that ECHA is acknowledged as the source: "Source: European Chemicals Agency, http://echa.europa.eu/". Such acknowledgement must be included in each copy of the material. ECHA permits and encourages organisations and individuals to create links to the ECHA website under the following cumulative conditions: Links can only be made to webpages that provide a link to the Legal Notice page. | |
Retrosynthesis Analysis
AI-Powered Synthesis Planning: Our tool employs the Template_relevance Pistachio, Template_relevance Bkms_metabolic, Template_relevance Pistachio_ringbreaker, Template_relevance Reaxys, Template_relevance Reaxys_biocatalysis model, leveraging a vast database of chemical reactions to predict feasible synthetic routes.
One-Step Synthesis Focus: Specifically designed for one-step synthesis, it provides concise and direct routes for your target compounds, streamlining the synthesis process.
Accurate Predictions: Utilizing the extensive PISTACHIO, BKMS_METABOLIC, PISTACHIO_RINGBREAKER, REAXYS, REAXYS_BIOCATALYSIS database, our tool offers high-accuracy predictions, reflecting the latest in chemical research and data.
Strategy Settings
Precursor scoring | Relevance Heuristic |
---|---|
Min. plausibility | 0.01 |
Model | Template_relevance |
Template Set | Pistachio/Bkms_metabolic/Pistachio_ringbreaker/Reaxys/Reaxys_biocatalysis |
Top-N result to add to graph | 6 |
Feasible Synthetic Routes
Disclaimer and Information on In-Vitro Research Products
Please be aware that all articles and product information presented on BenchChem are intended solely for informational purposes. The products available for purchase on BenchChem are specifically designed for in-vitro studies, which are conducted outside of living organisms. In-vitro studies, derived from the Latin term "in glass," involve experiments performed in controlled laboratory settings using cells or tissues. It is important to note that these products are not categorized as medicines or drugs, and they have not received approval from the FDA for the prevention, treatment, or cure of any medical condition, ailment, or disease. We must emphasize that any form of bodily introduction of these products into humans or animals is strictly prohibited by law. It is essential to adhere to these guidelines to ensure compliance with legal and ethical standards in research and experimentation.