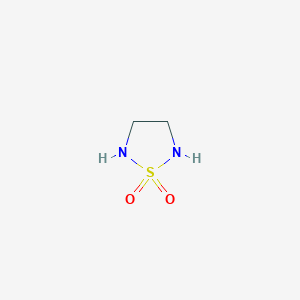
1,2,5-Thiadiazolidine 1,1-dioxide
Overview
Description
1,2,5-Thiadiazolidine 1,1-dioxide belongs to the class of organic compounds known as sulfanilides. These are organic aromatic compounds containing a sulfanilide moiety . It has been reported that 1,2,5-Thiadiazolidin-3-one 1,1-dioxide-based heterocyclic sulfides are potent inhibitors of human tryptase .
Synthesis Analysis
The synthesis of this compound involves several steps. Starting from proteogenic amino acids, the process includes reduction, carbamoylation-sulfamoylation, intermolecular cyclization via the Mitsunobu reaction, and acylation . Another method involves the reaction of sulfuryl chloride with 2-chloroethylamine or 3-chloropropylamine hydrochlorides, followed by treatment with a primary amine and triethylamine, and ring closure with K2CO3 in DMSO .
Molecular Structure Analysis
The molecular structure of this compound has been studied extensively. An overview of all currently known structures containing the 1,2,5-thiadiazole 1,1-dioxide motif (including the anions radical species) is provided according to the Cambridge Structural Database search . The analysis of the bond lengths typical for neutral and anion radical species is performed, providing a useful tool for unambiguous assessment of the valence state of the dioxothiadiazole-based compounds based solely on the structural data .
Chemical Reactions Analysis
The chemical reactions of this compound have been studied. The compound has been found to undergo various reactions, including mainly the chemical methods for the successful reduction of dioxothiadiazoles to their anion radical forms and the ability to form coordination compounds . Other reactions include the addition of alpha-diamines, such as ethylendiamine and o-phenylendiamine .
Physical And Chemical Properties Analysis
The physical and chemical properties of this compound have been studied. The compound is known for its reactivity, physicochemical properties, and main uses in chemistry and material sciences .
Scientific Research Applications
Enzyme Inhibition
1,2,5-Thiadiazolidine 1,1-dioxide has been developed as an inhibitor of the enzyme protein tyrosine phosphatase 1B (PTP1B), which is significant for its potential role in therapeutic interventions (Ruddraraju et al., 2015). Similarly, it has been investigated for its inhibitory activity towards human leukocyte elastase and cathepsin G, indicating its potential in therapeutic applications related to these enzymes (Groutas et al., 1994).
Chemical Reactions and Structure
The compound reacts interestingly with sulfuryl chloride, leading to unexpected dimeric products, showcasing its unique reactivity and structural properties (Dou et al., 2009). Another study focused on the synthesis and structure of chiral substituted 1,2,5-thiadiazolidine 1,1-dioxides, examining their potential as peptidomimetic scaffolds, which is valuable in drug design and synthesis (Bendjeddou et al., 2006).
Inhibitory Potency and Molecular Interactions
This compound has been utilized to create inhibitors that show selective and potent inhibition of various enzymes like human leukocyte elastase, cathepsin G, and proteinase 3. These studies highlight its effectiveness and specificity in inhibiting these enzymes, which is crucial for therapeutic applications (Groutas et al., 1998).
Applications in Molecular Modelling
Its derivatives have been designed as inhibitors for major histocompatibility complex class-II, a crucial component in immunology. The molecular modelling and synthesis of these derivatives demonstrate the compound's utility in designing molecules with specific biological targets (Ducry et al., 1999).
Spectroscopy and Density Functional Studies
New thiadiazole 1,1-dioxide compounds, including this compound derivatives, have been synthesized and characterized using various spectroscopic methods. These studies provide insights into their molecular structures and electronic properties, which are essential for understanding their reactivity and potential applications (Alpaslan et al., 2018).
Exploration of the Scaffold for Enzyme Inhibition
The 1,2,5-thiadiazolidin-3-one 1,1 dioxide scaffold has been extensively studied for its potential in probing the subsites of various serine proteases, demonstrating its versatility and significance in enzyme inhibition research (Groutas et al., 2001).
Safety and Hazards
Safety measures for handling 1,2,5-Thiadiazolidine 1,1-dioxide include avoiding dust formation, avoiding breathing mist, gas or vapours, avoiding contact with skin and eye, using personal protective equipment, wearing chemical impermeable gloves, ensuring adequate ventilation, and removing all sources of ignition . This chemical is considered hazardous by the 2012 OSHA Hazard Communication Standard .
properties
IUPAC Name |
1,2,5-thiadiazolidine 1,1-dioxide | |
---|---|---|
Source | PubChem | |
URL | https://pubchem.ncbi.nlm.nih.gov | |
Description | Data deposited in or computed by PubChem | |
InChI |
InChI=1S/C2H6N2O2S/c5-7(6)3-1-2-4-7/h3-4H,1-2H2 | |
Source | PubChem | |
URL | https://pubchem.ncbi.nlm.nih.gov | |
Description | Data deposited in or computed by PubChem | |
InChI Key |
ADBZIZGMAVRJPN-UHFFFAOYSA-N | |
Source | PubChem | |
URL | https://pubchem.ncbi.nlm.nih.gov | |
Description | Data deposited in or computed by PubChem | |
Canonical SMILES |
C1CNS(=O)(=O)N1 | |
Source | PubChem | |
URL | https://pubchem.ncbi.nlm.nih.gov | |
Description | Data deposited in or computed by PubChem | |
Molecular Formula |
C2H6N2O2S | |
Source | PubChem | |
URL | https://pubchem.ncbi.nlm.nih.gov | |
Description | Data deposited in or computed by PubChem | |
DSSTOX Substance ID |
DTXSID00440245 | |
Record name | 1,2,5-thiadiazolidine 1,1-dioxide | |
Source | EPA DSSTox | |
URL | https://comptox.epa.gov/dashboard/DTXSID00440245 | |
Description | DSSTox provides a high quality public chemistry resource for supporting improved predictive toxicology. | |
Molecular Weight |
122.15 g/mol | |
Source | PubChem | |
URL | https://pubchem.ncbi.nlm.nih.gov | |
Description | Data deposited in or computed by PubChem | |
CAS RN |
5823-51-8 | |
Record name | 1,2,5-thiadiazolidine 1,1-dioxide | |
Source | EPA DSSTox | |
URL | https://comptox.epa.gov/dashboard/DTXSID00440245 | |
Description | DSSTox provides a high quality public chemistry resource for supporting improved predictive toxicology. | |
Record name | 1lambda6,2,5-thiadiazolidine-1,1-dione | |
Source | European Chemicals Agency (ECHA) | |
URL | https://echa.europa.eu/information-on-chemicals | |
Description | The European Chemicals Agency (ECHA) is an agency of the European Union which is the driving force among regulatory authorities in implementing the EU's groundbreaking chemicals legislation for the benefit of human health and the environment as well as for innovation and competitiveness. | |
Explanation | Use of the information, documents and data from the ECHA website is subject to the terms and conditions of this Legal Notice, and subject to other binding limitations provided for under applicable law, the information, documents and data made available on the ECHA website may be reproduced, distributed and/or used, totally or in part, for non-commercial purposes provided that ECHA is acknowledged as the source: "Source: European Chemicals Agency, http://echa.europa.eu/". Such acknowledgement must be included in each copy of the material. ECHA permits and encourages organisations and individuals to create links to the ECHA website under the following cumulative conditions: Links can only be made to webpages that provide a link to the Legal Notice page. | |
Retrosynthesis Analysis
AI-Powered Synthesis Planning: Our tool employs the Template_relevance Pistachio, Template_relevance Bkms_metabolic, Template_relevance Pistachio_ringbreaker, Template_relevance Reaxys, Template_relevance Reaxys_biocatalysis model, leveraging a vast database of chemical reactions to predict feasible synthetic routes.
One-Step Synthesis Focus: Specifically designed for one-step synthesis, it provides concise and direct routes for your target compounds, streamlining the synthesis process.
Accurate Predictions: Utilizing the extensive PISTACHIO, BKMS_METABOLIC, PISTACHIO_RINGBREAKER, REAXYS, REAXYS_BIOCATALYSIS database, our tool offers high-accuracy predictions, reflecting the latest in chemical research and data.
Strategy Settings
Precursor scoring | Relevance Heuristic |
---|---|
Min. plausibility | 0.01 |
Model | Template_relevance |
Template Set | Pistachio/Bkms_metabolic/Pistachio_ringbreaker/Reaxys/Reaxys_biocatalysis |
Top-N result to add to graph | 6 |
Feasible Synthetic Routes
Q & A
A: 1,2,5-Thiadiazolidine 1,1-dioxide is a five-membered heterocyclic compound containing a sulfonyl group. Its molecular formula is C2H4N2O2S, and its molecular weight is 124.14 g/mol. [] While specific spectroscopic data varies based on substitutions, these compounds are commonly characterized by IR, NMR, and mass spectrometry. [, ]
A: The cyclic structure of 1,2,5-Thiadiazolidine 1,1-dioxides, particularly when substituted with chiral groups, allows them to mimic the conformational restrictions found in dipeptides. This property makes them valuable tools in peptidomimetic research. [, ]
A: Yes, several synthetic approaches have been developed. One common method involves reacting sulfamide with a suitable diamine, such as trimethylenediamine. [] Another method utilizes the reaction of α-amino acid esters with chlorosulfonyl isocyanate. [] More specialized methods employ the Mitsunobu reaction. [, ]
A: The electron-withdrawing nature of the sulfonyl group significantly influences the reactivity of the adjacent nitrogen atoms, impacting their nucleophilicity and making them less basic. [] This property can be exploited for various chemical transformations. For example, it facilitates the N-arylation of N5H-1,2,5-thiadiazolidine 1,1-dioxides using copper catalysts. []
A: Chiral centers within the molecule, often derived from starting amino acids, can significantly influence biological activity. The development of stereoselective syntheses is crucial for obtaining enantiomerically pure compounds. [, ] These chiral derivatives can be further converted into valuable chiral building blocks, such as unsymmetrical vicinal diamines. []
ANone: Yes, research indicates that certain derivatives exhibit biological activity:
- Antibacterial activity: Studies have investigated the antibacterial effects of 3-imino-4-substituted-1,2,5-thiadiazolidine 1,1-dioxides on various bacterial strains, including Bacillus subtilis, Escherichia coli, and Staphylococcus aureus. []
- Antiviral activity: Aminopyridyl this compound derivatives have shown promising antiviral activity against Enterovirus 71 (EV71), the causative agent of Hand, Foot, and Mouth Disease. []
- Enzyme inhibition: Substituted 3-oxo-1,2,5-thiadiazolidine 1,1-dioxides have demonstrated potential as mechanism-based inhibitors of human leukocyte elastase and cathepsin G. []
A: One notable limitation is the potential decrease in anticonvulsant activity when the central carbonyl group in hydantoins is replaced by a sulfonyl moiety, as seen in 3-oxo- and 3-imino-4-substituted 1,2,5-thiadiazolidine 1,1-dioxides. [] This highlights the importance of careful structural modifications and their impact on desired biological activities.
ANone: Future research could focus on:
A: Various analytical techniques are employed, including:* X-ray crystallography: Used to determine the crystal structure of synthesized compounds. [, ]* NMR spectroscopy (1H, 13C, 31P): Used to confirm the structure and purity of synthesized compounds. [, ]* Mass spectrometry: Employed to determine molecular weight and identify fragments. []* Infrared spectroscopy: Used to characterize functional groups present in the molecule. []
A: Yes, computational methods, like molecular modeling and docking studies, can help predict interactions with biological targets. Furthermore, QSAR (Quantitative Structure-Activity Relationship) studies can be conducted to analyze the relationship between the structure of this compound derivatives and their biological activities. [, , ] This information can guide the design of new and more potent derivatives.
Disclaimer and Information on In-Vitro Research Products
Please be aware that all articles and product information presented on BenchChem are intended solely for informational purposes. The products available for purchase on BenchChem are specifically designed for in-vitro studies, which are conducted outside of living organisms. In-vitro studies, derived from the Latin term "in glass," involve experiments performed in controlled laboratory settings using cells or tissues. It is important to note that these products are not categorized as medicines or drugs, and they have not received approval from the FDA for the prevention, treatment, or cure of any medical condition, ailment, or disease. We must emphasize that any form of bodily introduction of these products into humans or animals is strictly prohibited by law. It is essential to adhere to these guidelines to ensure compliance with legal and ethical standards in research and experimentation.