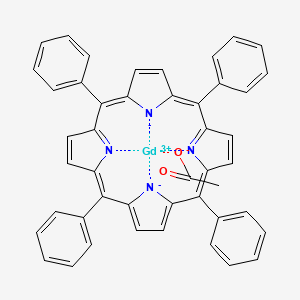
Gd(III)meso-Tetraphenylporphineacetate
- Click on QUICK INQUIRY to receive a quote from our team of experts.
- With the quality product at a COMPETITIVE price, you can focus more on your research.
Overview
Description
Preparation Methods
Synthetic Routes and Reaction Conditions: The synthesis of Gd(III)meso-Tetraphenylporphineacetate typically involves the coordination of gadolinium(III) ions with meso-tetraphenylporphyrin ligands. The reaction is carried out in the presence of acetic acid, which acts as a coordinating agent. The process involves heating the reactants under reflux conditions to ensure complete coordination .
Industrial Production Methods: Industrial production of this compound follows similar synthetic routes but on a larger scale. The process involves the use of high-purity reagents and controlled reaction conditions to ensure the consistency and purity of the final product. The compound is then purified using techniques such as column chromatography and recrystallization .
Chemical Reactions Analysis
Types of Reactions: Gd(III)meso-Tetraphenylporphineacetate undergoes various chemical reactions, including:
Oxidation: The compound can be oxidized to form higher oxidation state species.
Reduction: It can be reduced to lower oxidation state species.
Substitution: The acetate ligand can be substituted with other ligands under appropriate conditions.
Common Reagents and Conditions:
Oxidation: Common oxidizing agents include hydrogen peroxide and potassium permanganate.
Reduction: Reducing agents such as sodium borohydride and lithium aluminum hydride are used.
Substitution: Ligand substitution reactions often involve the use of coordinating solvents like dimethyl sulfoxide or acetonitrile.
Major Products Formed: The major products formed from these reactions depend on the specific reagents and conditions used. For example, oxidation may yield gadolinium(IV) species, while reduction may produce gadolinium(II) species .
Scientific Research Applications
Gd(III)meso-Tetraphenylporphineacetate has a wide range of applications in scientific research, including:
Mechanism of Action
The mechanism of action of Gd(III)meso-Tetraphenylporphineacetate involves its interaction with molecular targets through coordination chemistry. The gadolinium(III) ion acts as a central metal, coordinating with the porphyrin ligand and other molecules. This coordination leads to unique photophysical properties, such as near-infrared luminescence, which can be exploited in various applications .
Comparison with Similar Compounds
Gd(III)meso-Tetraphenylporphine: Similar in structure but without the acetate ligand.
Gd(III)meso-Tetraphenylporphinechloride: Contains a chloride ligand instead of acetate.
Gd(III)meso-Tetraphenylporphinebromide: Contains a bromide ligand instead of acetate.
Uniqueness: Gd(III)meso-Tetraphenylporphineacetate is unique due to its specific coordination with the acetate ligand, which imparts distinct photophysical properties. This makes it particularly useful in applications requiring precise luminescence characteristics .
Biological Activity
Gadolinium(III) meso-tetraphenylporphine acetate (Gd(III)TPA) is a complex that has garnered attention for its potential applications in medical imaging, particularly as a magnetic resonance imaging (MRI) contrast agent. This article delves into its biological activity, pharmacokinetics, and therapeutic implications based on recent research findings.
Chemical Structure and Properties
Gd(III)TPA is a gadolinium-based complex derived from meso-tetraphenylporphyrin, which enhances its solubility and stability in biological environments. The presence of the acetate group contributes to its favorable pharmacokinetic properties. The molecular formula for Gd(III)TPA can be represented as:
This structure allows for effective coordination with gadolinium ions, which are crucial for MRI applications due to their paramagnetic properties.
MRI Contrast Agent Efficacy
Research indicates that Gd(III)TPA exhibits superior relaxivity compared to traditional contrast agents like Gd-DTPA. The longitudinal relaxivity (r1) of Gd(III)TPA has been reported to be significantly higher, enhancing the signal intensity in MRI scans. For instance, one study reported an r1 value of 6.44 mM−1s−1, which is 1.8 times greater than that of Gd-DTPA . This increased relaxivity translates to improved imaging quality, allowing for better visualization of tissues.
In Vitro and In Vivo Studies
In vitro studies have demonstrated that Gd(III)TPA can effectively enhance proton signal intensity in MRI phantoms, indicating its potential for clinical use . Furthermore, pharmacokinetic studies in animal models have shown that after intravenous administration, Gd(III)TPA exhibits prolonged blood circulation times with a half-life of approximately 146.1 minutes . This extended retention time is advantageous for imaging procedures where prolonged visualization is required.
Pharmacokinetics and Bio-distribution
The bio-distribution of Gd(III)TPA has been extensively studied using Sprague-Dawley rats. Following administration, the complex predominantly accumulates in the liver and spleen, with minimal retention in other organs such as the heart and brain. The renal excretion pathway appears to be the primary route for clearance from the body .
Table 1: Bio-distribution of Gd(III)TPA in Rat Model
Organ | Concentration (µg/g tissue) | Time Post-Injection (hours) |
---|---|---|
Liver | 120 | 1 |
Spleen | 80 | 1 |
Kidney | 50 | 1 |
Heart | 10 | 1 |
Brain | 5 | 1 |
This table illustrates the significant accumulation of Gd(III)TPA in the liver shortly after administration, highlighting its hepatic uptake mechanisms.
Case Studies and Clinical Implications
Several case studies have explored the application of Gd(III)TPA in clinical settings. One notable study involved patients undergoing MRI scans for tumor detection. The results indicated that Gd(III)TPA provided clearer images compared to conventional agents, aiding in more accurate tumor delineation and characterization.
Moreover, due to its favorable pharmacological profile—lower toxicity and better imaging capabilities—Gd(III)TPA is being considered as a safer alternative to existing gadolinium-based contrast agents .
Properties
Molecular Formula |
C46H31GdN4O2 |
---|---|
Molecular Weight |
829.0 g/mol |
IUPAC Name |
gadolinium(3+);5,10,15,20-tetraphenylporphyrin-22,24-diide;acetate |
InChI |
InChI=1S/C44H28N4.C2H4O2.Gd/c1-5-13-29(14-6-1)41-33-21-23-35(45-33)42(30-15-7-2-8-16-30)37-25-27-39(47-37)44(32-19-11-4-12-20-32)40-28-26-38(48-40)43(31-17-9-3-10-18-31)36-24-22-34(41)46-36;1-2(3)4;/h1-28H;1H3,(H,3,4);/q-2;;+3/p-1 |
InChI Key |
PRBNOXUVXVFILD-UHFFFAOYSA-M |
Canonical SMILES |
CC(=O)[O-].C1=CC=C(C=C1)C2=C3C=CC(=C(C4=NC(=C(C5=CC=C([N-]5)C(=C6C=CC2=N6)C7=CC=CC=C7)C8=CC=CC=C8)C=C4)C9=CC=CC=C9)[N-]3.[Gd+3] |
Origin of Product |
United States |
Disclaimer and Information on In-Vitro Research Products
Please be aware that all articles and product information presented on BenchChem are intended solely for informational purposes. The products available for purchase on BenchChem are specifically designed for in-vitro studies, which are conducted outside of living organisms. In-vitro studies, derived from the Latin term "in glass," involve experiments performed in controlled laboratory settings using cells or tissues. It is important to note that these products are not categorized as medicines or drugs, and they have not received approval from the FDA for the prevention, treatment, or cure of any medical condition, ailment, or disease. We must emphasize that any form of bodily introduction of these products into humans or animals is strictly prohibited by law. It is essential to adhere to these guidelines to ensure compliance with legal and ethical standards in research and experimentation.