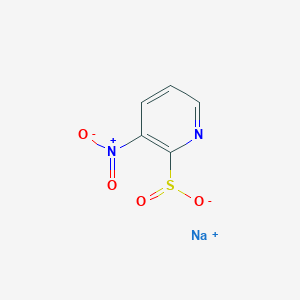
Sodium 3-nitropyridine-2-sulfinate
- Click on QUICK INQUIRY to receive a quote from our team of experts.
- With the quality product at a COMPETITIVE price, you can focus more on your research.
Overview
Description
Sodium 3-nitropyridine-2-sulfinate is an organosulfur compound that has gained attention in recent years due to its versatile reactivity and potential applications in various fields. This compound is a sodium salt of 3-nitropyridine-2-sulfinic acid and is characterized by its stability and ease of handling compared to its parent sulfinic acid.
Preparation Methods
Synthetic Routes and Reaction Conditions: The synthesis of sodium 3-nitropyridine-2-sulfinate typically involves the reaction of 3-nitropyridine with sodium sulfite. The reaction is carried out in an aqueous medium, and the product is isolated by crystallization. The general reaction can be represented as follows: [ \text{3-nitropyridine} + \text{Na}_2\text{SO}_3 \rightarrow \text{this compound} ]
Industrial Production Methods: Industrial production of this compound follows similar synthetic routes but on a larger scale. The reaction conditions are optimized to ensure high yield and purity of the product. The use of continuous flow reactors and automated crystallization techniques are common in industrial settings to enhance efficiency and scalability.
Chemical Reactions Analysis
Types of Reactions: Sodium 3-nitropyridine-2-sulfinate undergoes various chemical reactions, including:
Oxidation: It can be oxidized to form sulfonic acids.
Reduction: Reduction reactions can convert it to the corresponding amine.
Substitution: It can participate in nucleophilic substitution reactions, where the sulfinate group is replaced by other nucleophiles.
Common Reagents and Conditions:
Oxidation: Common oxidizing agents include hydrogen peroxide and potassium permanganate.
Reduction: Reducing agents such as sodium borohydride or catalytic hydrogenation are used.
Substitution: Reagents like alkyl halides or acyl chlorides can be used under basic conditions.
Major Products Formed:
Oxidation: 3-nitropyridine-2-sulfonic acid.
Reduction: 3-aminopyridine.
Substitution: Various substituted pyridines depending on the nucleophile used.
Scientific Research Applications
Sodium 3-nitropyridine-2-sulfinate has found applications in several scientific research areas:
Chemistry: It is used as a building block for the synthesis of more complex organosulfur compounds.
Biology: Its derivatives are studied for their potential biological activities, including antimicrobial and anticancer properties.
Medicine: Research is ongoing to explore its use in drug development, particularly in designing molecules with improved pharmacokinetic properties.
Industry: It is used in the production of dyes, pigments, and other specialty chemicals.
Mechanism of Action
The mechanism by which sodium 3-nitropyridine-2-sulfinate exerts its effects involves the formation of sulfonyl radicals under specific conditions. These radicals can participate in various chemical transformations, including radical addition and substitution reactions. The molecular targets and pathways involved depend on the specific application and reaction conditions.
Comparison with Similar Compounds
- Sodium nitrobenzenesulfinate
- Sodium trifluoromethanesulfinate
- Sodium thiophene-2-sulfinate
- Sodium pyridine-3-sulfinate
Comparison: Sodium 3-nitropyridine-2-sulfinate is unique due to its nitro-substituted pyridine ring, which imparts distinct reactivity compared to other sulfinates. For instance, sodium trifluoromethanesulfinate is more commonly used in trifluoromethylation reactions, while sodium thiophene-2-sulfinate is preferred for thiophene-based syntheses. The nitro group in this compound enhances its electrophilicity, making it a valuable reagent in nucleophilic substitution reactions .
Biological Activity
Sodium 3-nitropyridine-2-sulfinate is an organosulfur compound that has garnered attention for its potential biological activities, particularly in antimicrobial and anticancer contexts. This article reviews the current understanding of its biological activity, mechanisms of action, and relevant research findings.
Chemical Structure and Properties
This compound is characterized by the following structural features:
- Molecular Formula : C6H6N2O3S
- Molecular Weight : Approximately 210.15 g/mol
- Functional Groups : Contains a nitro group (-NO2) at the 3-position and a sulfinate group (-SO2−) at the 2-position of the pyridine ring.
The presence of these functional groups contributes to its unique reactivity and biological activity, particularly through sulfonylation reactions that can modify biomolecules.
Antimicrobial Properties
Research indicates that this compound exhibits significant antimicrobial properties. The nitro group in its structure plays a critical role in enhancing its antibacterial activity. Studies have shown that compounds with similar nitro groups demonstrate toxicity towards various microorganisms, including bacteria and parasites, by triggering redox reactions that lead to cell death .
Table 1: Antimicrobial Activity of this compound
Microorganism | Minimum Inhibitory Concentration (MIC) | Reference |
---|---|---|
Staphylococcus aureus | 20 μM | |
Pseudomonas aeruginosa | 30 μM | |
Mycobacterium tuberculosis | 0.78 μM |
The antimicrobial mechanism is believed to involve the formation of reactive intermediates that can interact with essential cellular components, leading to cell lysis and death.
Anticancer Activity
This compound has also been studied for its potential anticancer properties. The sulfonylating nature of this compound allows it to modify thiol groups in proteins, which could alter their function and activity, potentially leading to apoptosis in cancer cells.
Research has indicated that compounds containing nitro groups can act as both pharmacophores (active therapeutic agents) and toxicophores (agents causing toxicity), suggesting a dual role in cancer therapy by targeting tumor cells while minimizing effects on normal cells .
The biological activity of this compound is primarily attributed to its ability to form sulfonyl radicals under specific conditions. These radicals can undergo various chemical transformations, including:
- Radical Addition : Involves the addition of sulfonyl radicals to double bonds in biomolecules.
- Nucleophilic Substitution : The sulfinate group can be replaced by other nucleophiles, modifying the target molecule's structure and function.
These reactions suggest that this compound may influence multiple biochemical pathways, enhancing its therapeutic potential .
Case Studies
Several studies have explored the biological activities of this compound:
- Antimicrobial Efficacy : A study demonstrated its effectiveness against resistant strains of bacteria, highlighting its potential as a new antimicrobial agent in clinical settings.
- Cancer Cell Line Studies : Research involving various cancer cell lines showed that this compound induced apoptosis through oxidative stress mechanisms, suggesting a promising avenue for cancer treatment.
Properties
Molecular Formula |
C5H3N2NaO4S |
---|---|
Molecular Weight |
210.15 g/mol |
IUPAC Name |
sodium;3-nitropyridine-2-sulfinate |
InChI |
InChI=1S/C5H4N2O4S.Na/c8-7(9)4-2-1-3-6-5(4)12(10)11;/h1-3H,(H,10,11);/q;+1/p-1 |
InChI Key |
KIVQIFTUBKUBOS-UHFFFAOYSA-M |
Canonical SMILES |
C1=CC(=C(N=C1)S(=O)[O-])[N+](=O)[O-].[Na+] |
Origin of Product |
United States |
Disclaimer and Information on In-Vitro Research Products
Please be aware that all articles and product information presented on BenchChem are intended solely for informational purposes. The products available for purchase on BenchChem are specifically designed for in-vitro studies, which are conducted outside of living organisms. In-vitro studies, derived from the Latin term "in glass," involve experiments performed in controlled laboratory settings using cells or tissues. It is important to note that these products are not categorized as medicines or drugs, and they have not received approval from the FDA for the prevention, treatment, or cure of any medical condition, ailment, or disease. We must emphasize that any form of bodily introduction of these products into humans or animals is strictly prohibited by law. It is essential to adhere to these guidelines to ensure compliance with legal and ethical standards in research and experimentation.