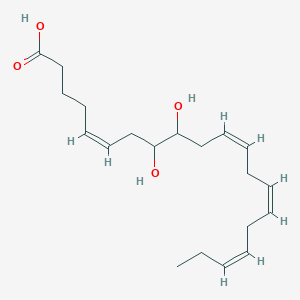
(+/-)-8,9-dihydroxy-5Z,11Z,14Z,17Z-eicosatetraenoic acid
Overview
Description
8,9-DiHETE is a major metabolite of the 20:5 ω-3 fatty acid eicosapentaenoic acid (EPA; ). It is produced in rat liver microsomes, but not renal microsomes, by the generation of the unstable intermediate 8,9-epoxy eicosatetraenoic acid from EPA by cytochrome P450 monooxygenases. Dietary EPA supplementation in humans results in substantial urinary excretion of vicinal diols, including 8,9, 11,12, and 14,15 forms.
Mechanism of Action
Target of Action
The primary targets of 8,9-DiHETE are cytochrome P450 (P450) enzymes . These enzymes play a crucial role in the metabolism of arachidonic acid (AA), generating eicosanoids that serve as important autocrine and paracrine factors with diverse biological functions .
Mode of Action
8,9-DiHETE is an oxylipin, a class of signaling molecules derived from the oxidation of fatty acids. It is a racemic mixture of the arachidonic acid metabolites 8(S),9®-DiHET and 8®,9(S)-DiHET . It is formed from arachidonic acid via an (±)8(9)-EET intermediate by epoxide hydrolases .
Biochemical Pathways
8,9-DiHETE is involved in the cytochrome P450 pathways . It is a major metabolite of the 20:5 ω-3 fatty acid eicosapentaenoic acid (EPA) and is produced in rat liver microsomes by the generation of the unstable intermediate 8,9-epoxy eicosatetraenoic acid from EPA by cytochrome P450 monooxygenases .
Pharmacokinetics
It is known that dietary epa supplementation in humans results in substantial urinary excretion of vicinal diols, including 8,9-dihete . This suggests that the compound is well-absorbed and metabolized, and its bioavailability is influenced by dietary intake of EPA.
Result of Action
8,9-DiHETE has been shown to induce cAMP production in primary human coronary artery smooth muscle cells . This suggests that it may play a role in regulating vascular tone. Additionally, plasma levels of 8,9-DiHETE are increased in a mouse model of osteoarthritic pain induced by destabilization of the medial meniscus . This effect can be reversed by the soluble epoxide hydrolase (sEH) inhibitor TPPU .
Biochemical Analysis
Biochemical Properties
8,9-DiHETE plays a significant role in biochemical reactions. It interacts with various enzymes, proteins, and other biomolecules. For instance, it is formed from arachidonic acid via an 8,9-EET intermediate by epoxide hydrolases . The nature of these interactions involves the conversion of EETs into vicinal diols, with the concurrent loss of much of their biological activity .
Cellular Effects
8,9-DiHETE has profound effects on various types of cells and cellular processes. It influences cell function, including impacts on cell signaling pathways, gene expression, and cellular metabolism. For example, 8,9-DiHETE (1 µM) induces cAMP production in primary human coronary artery smooth muscle cells .
Molecular Mechanism
The molecular mechanism of 8,9-DiHETE involves its role as a signaling molecule. It exerts its effects at the molecular level, including binding interactions with biomolecules, enzyme inhibition or activation, and changes in gene expression. It is formed from arachidonic acid via an 8,9-EET intermediate by epoxide hydrolases .
Metabolic Pathways
8,9-DiHETE is involved in several metabolic pathways. It interacts with enzymes or cofactors in these pathways, which could also include any effects on metabolic flux or metabolite levels. For instance, it is a part of the Cytochrome P450 (P450) eicosanoid pathway .
Properties
IUPAC Name |
(5Z,11Z,14Z,17Z)-8,9-dihydroxyicosa-5,11,14,17-tetraenoic acid | |
---|---|---|
Source | PubChem | |
URL | https://pubchem.ncbi.nlm.nih.gov | |
Description | Data deposited in or computed by PubChem | |
InChI |
InChI=1S/C20H32O4/c1-2-3-4-5-6-7-8-9-12-15-18(21)19(22)16-13-10-11-14-17-20(23)24/h3-4,6-7,9-10,12-13,18-19,21-22H,2,5,8,11,14-17H2,1H3,(H,23,24)/b4-3-,7-6-,12-9-,13-10- | |
Source | PubChem | |
URL | https://pubchem.ncbi.nlm.nih.gov | |
Description | Data deposited in or computed by PubChem | |
InChI Key |
NXFSSCYFERVGJQ-JJUYGIQRSA-N | |
Source | PubChem | |
URL | https://pubchem.ncbi.nlm.nih.gov | |
Description | Data deposited in or computed by PubChem | |
Canonical SMILES |
CCC=CCC=CCC=CCC(C(CC=CCCCC(=O)O)O)O | |
Source | PubChem | |
URL | https://pubchem.ncbi.nlm.nih.gov | |
Description | Data deposited in or computed by PubChem | |
Isomeric SMILES |
CC/C=C\C/C=C\C/C=C\CC(C(C/C=C\CCCC(=O)O)O)O | |
Source | PubChem | |
URL | https://pubchem.ncbi.nlm.nih.gov | |
Description | Data deposited in or computed by PubChem | |
Molecular Formula |
C20H32O4 | |
Source | PubChem | |
URL | https://pubchem.ncbi.nlm.nih.gov | |
Description | Data deposited in or computed by PubChem | |
DSSTOX Substance ID |
DTXSID201348077 | |
Record name | (5Z,11Z,14Z,17Z)-8,9-Dihydroxy-5,11,14,17-icosatetraenoic acid | |
Source | EPA DSSTox | |
URL | https://comptox.epa.gov/dashboard/DTXSID201348077 | |
Description | DSSTox provides a high quality public chemistry resource for supporting improved predictive toxicology. | |
Molecular Weight |
336.5 g/mol | |
Source | PubChem | |
URL | https://pubchem.ncbi.nlm.nih.gov | |
Description | Data deposited in or computed by PubChem | |
CAS No. |
867350-87-6 | |
Record name | (5Z,11Z,14Z,17Z)-8,9-Dihydroxy-5,11,14,17-icosatetraenoic acid | |
Source | EPA DSSTox | |
URL | https://comptox.epa.gov/dashboard/DTXSID201348077 | |
Description | DSSTox provides a high quality public chemistry resource for supporting improved predictive toxicology. | |
Retrosynthesis Analysis
AI-Powered Synthesis Planning: Our tool employs the Template_relevance Pistachio, Template_relevance Bkms_metabolic, Template_relevance Pistachio_ringbreaker, Template_relevance Reaxys, Template_relevance Reaxys_biocatalysis model, leveraging a vast database of chemical reactions to predict feasible synthetic routes.
One-Step Synthesis Focus: Specifically designed for one-step synthesis, it provides concise and direct routes for your target compounds, streamlining the synthesis process.
Accurate Predictions: Utilizing the extensive PISTACHIO, BKMS_METABOLIC, PISTACHIO_RINGBREAKER, REAXYS, REAXYS_BIOCATALYSIS database, our tool offers high-accuracy predictions, reflecting the latest in chemical research and data.
Strategy Settings
Precursor scoring | Relevance Heuristic |
---|---|
Min. plausibility | 0.01 |
Model | Template_relevance |
Template Set | Pistachio/Bkms_metabolic/Pistachio_ringbreaker/Reaxys/Reaxys_biocatalysis |
Top-N result to add to graph | 6 |
Feasible Synthetic Routes
Disclaimer and Information on In-Vitro Research Products
Please be aware that all articles and product information presented on BenchChem are intended solely for informational purposes. The products available for purchase on BenchChem are specifically designed for in-vitro studies, which are conducted outside of living organisms. In-vitro studies, derived from the Latin term "in glass," involve experiments performed in controlled laboratory settings using cells or tissues. It is important to note that these products are not categorized as medicines or drugs, and they have not received approval from the FDA for the prevention, treatment, or cure of any medical condition, ailment, or disease. We must emphasize that any form of bodily introduction of these products into humans or animals is strictly prohibited by law. It is essential to adhere to these guidelines to ensure compliance with legal and ethical standards in research and experimentation.