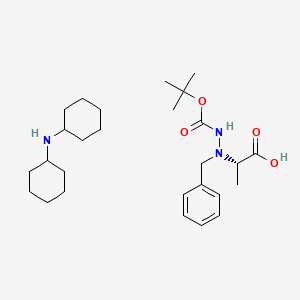
Dicyclohexylamine (S)-2-(1-benzyl-2-(tert-butoxycarbonyl)hydrazinyl)propanoate
- Click on QUICK INQUIRY to receive a quote from our team of experts.
- With the quality product at a COMPETITIVE price, you can focus more on your research.
Overview
Description
Dicyclohexylamine (S)-2-(1-benzyl-2-(tert-butoxycarbonyl)hydrazinyl)propanoate is a complex organic compound that features a tert-butoxycarbonyl (Boc) protecting group. This compound is of interest in various fields of chemistry and biology due to its unique structural properties and reactivity patterns.
Preparation Methods
Synthetic Routes and Reaction Conditions
The synthesis of Dicyclohexylamine (S)-2-(1-benzyl-2-(tert-butoxycarbonyl)hydrazinyl)propanoate typically involves multiple steps, starting from readily available starting materials. The key steps include:
Formation of the hydrazine derivative: This involves the reaction of a benzyl hydrazine with a suitable carboxylic acid derivative.
Introduction of the Boc protecting group: The tert-butoxycarbonyl group is introduced using di-tert-butyl dicarbonate (Boc2O) under basic conditions.
Coupling with Dicyclohexylamine: The final step involves coupling the protected hydrazine derivative with dicyclohexylamine under appropriate conditions to yield the target compound.
Industrial Production Methods
Industrial production of this compound would likely follow similar synthetic routes but on a larger scale, with optimizations for yield, purity, and cost-effectiveness. This might involve the use of continuous flow reactors and automated synthesis equipment to ensure consistent quality and efficiency.
Chemical Reactions Analysis
Types of Reactions
Dicyclohexylamine (S)-2-(1-benzyl-2-(tert-butoxycarbonyl)hydrazinyl)propanoate can undergo various types of chemical reactions, including:
Oxidation: The compound can be oxidized under specific conditions to form corresponding oxides.
Reduction: Reduction reactions can be carried out to remove the Boc protecting group, revealing the free hydrazine.
Substitution: The compound can participate in nucleophilic substitution reactions, where the Boc group can be replaced by other functional groups.
Common Reagents and Conditions
Oxidation: Common oxidizing agents include potassium permanganate (KMnO4) and chromium trioxide (CrO3).
Reduction: Reducing agents such as lithium aluminum hydride (LiAlH4) or hydrogen gas (H2) in the presence of a palladium catalyst can be used.
Substitution: Nucleophilic substitution reactions often involve reagents like sodium hydride (NaH) or other strong bases.
Major Products
The major products formed from these reactions depend on the specific conditions and reagents used. For example, oxidation might yield oxides, while reduction would yield the deprotected hydrazine derivative.
Scientific Research Applications
Dicyclohexylamine (S)-2-(1-benzyl-2-(tert-butoxycarbonyl)hydrazinyl)propanoate has several applications in scientific research:
Chemistry: It is used as an intermediate in the synthesis of more complex molecules, particularly in the field of organic synthesis.
Biology: The compound can be used in the study of enzyme mechanisms and as a probe in biochemical assays.
Industry: The compound can be used in the production of specialty chemicals and materials.
Mechanism of Action
The mechanism of action of Dicyclohexylamine (S)-2-(1-benzyl-2-(tert-butoxycarbonyl)hydrazinyl)propanoate involves its interaction with specific molecular targets. The Boc protecting group can be selectively removed under acidic conditions, revealing the active hydrazine moiety. This hydrazine can then interact with various biological targets, including enzymes and receptors, to exert its effects.
Comparison with Similar Compounds
Similar Compounds
Dicyclohexylamine (S)-2-(1-benzyl-2-(tert-butoxycarbonyl)hydrazinyl)butanoate: Similar structure but with a butanoate group instead of a propanoate group.
Dicyclohexylamine (S)-2-(1-benzyl-2-(tert-butoxycarbonyl)hydrazinyl)acetate: Similar structure but with an acetate group instead of a propanoate group.
Uniqueness
The uniqueness of Dicyclohexylamine (S)-2-(1-benzyl-2-(tert-butoxycarbonyl)hydrazinyl)propanoate lies in its specific combination of functional groups, which confer distinct reactivity and stability properties. The presence of the Boc protecting group allows for selective deprotection, making it a valuable intermediate in synthetic chemistry.
Properties
Molecular Formula |
C27H45N3O4 |
---|---|
Molecular Weight |
475.7 g/mol |
IUPAC Name |
(2S)-2-[benzyl-[(2-methylpropan-2-yl)oxycarbonylamino]amino]propanoic acid;N-cyclohexylcyclohexanamine |
InChI |
InChI=1S/C15H22N2O4.C12H23N/c1-11(13(18)19)17(10-12-8-6-5-7-9-12)16-14(20)21-15(2,3)4;1-3-7-11(8-4-1)13-12-9-5-2-6-10-12/h5-9,11H,10H2,1-4H3,(H,16,20)(H,18,19);11-13H,1-10H2/t11-;/m0./s1 |
InChI Key |
MDGLAMPROYHLDD-MERQFXBCSA-N |
Isomeric SMILES |
C[C@@H](C(=O)O)N(CC1=CC=CC=C1)NC(=O)OC(C)(C)C.C1CCC(CC1)NC2CCCCC2 |
Canonical SMILES |
CC(C(=O)O)N(CC1=CC=CC=C1)NC(=O)OC(C)(C)C.C1CCC(CC1)NC2CCCCC2 |
Origin of Product |
United States |
Disclaimer and Information on In-Vitro Research Products
Please be aware that all articles and product information presented on BenchChem are intended solely for informational purposes. The products available for purchase on BenchChem are specifically designed for in-vitro studies, which are conducted outside of living organisms. In-vitro studies, derived from the Latin term "in glass," involve experiments performed in controlled laboratory settings using cells or tissues. It is important to note that these products are not categorized as medicines or drugs, and they have not received approval from the FDA for the prevention, treatment, or cure of any medical condition, ailment, or disease. We must emphasize that any form of bodily introduction of these products into humans or animals is strictly prohibited by law. It is essential to adhere to these guidelines to ensure compliance with legal and ethical standards in research and experimentation.