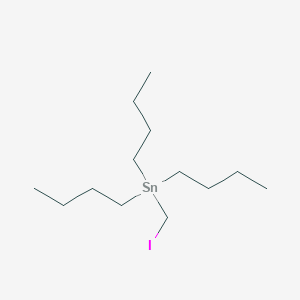
Tributyl(iodomethyl)stannane
Overview
Description
Tributyl(iodomethyl)stannane, also known as Stannane, tributyl(iodomethyl)-, is an organotin compound with the molecular formula C13H29ISn . It has an average mass of 430.984 Da and a mono-isotopic mass of 432.033600 Da .
Molecular Structure Analysis
The molecular structure of Tributyl(iodomethyl)stannane consists of 13 carbon atoms, 29 hydrogen atoms, 1 iodine atom, and 1 tin atom .Physical And Chemical Properties Analysis
Tributyl(iodomethyl)stannane has a boiling point of 337.3±44.0 °C at 760 mmHg. It has a vapour pressure of 0.0±0.7 mmHg at 25°C and an enthalpy of vaporization of 55.7±3.0 kJ/mol . The flash point is 157.8±28.4 °C .Scientific Research Applications
Organic Synthesis
Tributyl(iodomethyl)stannane is a valuable reagent in organic synthesis, particularly for the preparation of precursors that generate more reactive nucleophiles. These nucleophiles are used in [2,3]-sigmatropic Wittig rearrangements or can react further with other electrophiles such as ketones .
Radical Reactions
This compound is also utilized in standard radical reactions as a replacement for other organotin hydrides. The tin-containing byproducts from these reactions can be removed through mild hydrolysis and extraction with aqueous NaHCO3, simplifying the purification process .
Mechanism of Action
Target of Action
Tributyl(iodomethyl)stannane, also known as Tributylstannyliodomethane , is a type of organotin compoundOrganotin compounds are generally known for their ability to interact with various organic compounds, particularly those containing halides and selenides .
Mode of Action
Tributyl(iodomethyl)stannane is known to participate in radical reactions . It acts as a reducing agent, capable of cleaving relatively weak, nonionic bonds due to the presence of tin . This compound can replace other stannanes like Bu3SnH and Ph3SnH in standard radical reactions . The tin-containing byproducts from these reactions are removed by mild hydrolysis and extraction with aqueous NaHCO3 .
Biochemical Pathways
Organotin compounds like this are known to be involved in various reactions such as the barton-mccombie reaction, barton decarboxylation, dehalogenation, and intramolecular radical cyclization .
Pharmacokinetics
Organotin compounds are generally known for their high toxicity and lipophilicity , which could influence their absorption and distribution within the body.
Action Environment
The performance of organotin compounds in reactions can be influenced by various factors, including the presence of other reagents and the conditions under which the reactions are carried out .
properties
IUPAC Name |
tributyl(iodomethyl)stannane | |
---|---|---|
Source | PubChem | |
URL | https://pubchem.ncbi.nlm.nih.gov | |
Description | Data deposited in or computed by PubChem | |
InChI |
InChI=1S/3C4H9.CH2I.Sn/c3*1-3-4-2;1-2;/h3*1,3-4H2,2H3;1H2; | |
Source | PubChem | |
URL | https://pubchem.ncbi.nlm.nih.gov | |
Description | Data deposited in or computed by PubChem | |
InChI Key |
XEKIDGIZQAEOKB-UHFFFAOYSA-N | |
Source | PubChem | |
URL | https://pubchem.ncbi.nlm.nih.gov | |
Description | Data deposited in or computed by PubChem | |
Canonical SMILES |
CCCC[Sn](CCCC)(CCCC)CI | |
Source | PubChem | |
URL | https://pubchem.ncbi.nlm.nih.gov | |
Description | Data deposited in or computed by PubChem | |
Molecular Formula |
C13H29ISn | |
Source | PubChem | |
URL | https://pubchem.ncbi.nlm.nih.gov | |
Description | Data deposited in or computed by PubChem | |
DSSTOX Substance ID |
DTXSID60446263 | |
Record name | Stannane, tributyl(iodomethyl)- | |
Source | EPA DSSTox | |
URL | https://comptox.epa.gov/dashboard/DTXSID60446263 | |
Description | DSSTox provides a high quality public chemistry resource for supporting improved predictive toxicology. | |
Molecular Weight |
430.98 g/mol | |
Source | PubChem | |
URL | https://pubchem.ncbi.nlm.nih.gov | |
Description | Data deposited in or computed by PubChem | |
CAS RN |
66222-29-5 | |
Record name | Stannane, tributyl(iodomethyl)- | |
Source | EPA DSSTox | |
URL | https://comptox.epa.gov/dashboard/DTXSID60446263 | |
Description | DSSTox provides a high quality public chemistry resource for supporting improved predictive toxicology. | |
Record name | tributyl(iodomethyl)stannane | |
Source | European Chemicals Agency (ECHA) | |
URL | https://echa.europa.eu/information-on-chemicals | |
Description | The European Chemicals Agency (ECHA) is an agency of the European Union which is the driving force among regulatory authorities in implementing the EU's groundbreaking chemicals legislation for the benefit of human health and the environment as well as for innovation and competitiveness. | |
Explanation | Use of the information, documents and data from the ECHA website is subject to the terms and conditions of this Legal Notice, and subject to other binding limitations provided for under applicable law, the information, documents and data made available on the ECHA website may be reproduced, distributed and/or used, totally or in part, for non-commercial purposes provided that ECHA is acknowledged as the source: "Source: European Chemicals Agency, http://echa.europa.eu/". Such acknowledgement must be included in each copy of the material. ECHA permits and encourages organisations and individuals to create links to the ECHA website under the following cumulative conditions: Links can only be made to webpages that provide a link to the Legal Notice page. | |
Synthesis routes and methods
Procedure details
Q & A
Q1: How is Tributyl(iodomethyl)stannane utilized in synthesizing complex molecules?
A1: Tributyl(iodomethyl)stannane acts as a reagent in Stille cross-coupling reactions, facilitating the formation of new carbon-carbon bonds. [, ] This reaction is particularly useful in synthesizing analogues of pharmaceutical compounds, as demonstrated by its application in creating Doravirine analogues, a potential HIV NNRTI. [] This method provides a practical route to introduce diverse aryl groups onto the target molecule.
Q2: Can you elaborate on the specific advantages of using Tributyl(iodomethyl)stannane in synthesizing substituted thiomorpholines?
A2: Tributyl(iodomethyl)stannane offers a unique approach to synthesizing N-unprotected, 3-substituted thiomorpholines. [] Traditional methods for constructing such heterocycles often involve laborious procedures and may not be compatible with sensitive functional groups. Tributyl(iodomethyl)stannane, via the SnAP reagent strategy, utilizes a radical cyclization approach. This method employs readily available aldehydes as starting materials and provides a direct route to the desired N-unprotected thiomorpholines. []
Q3: What are the limitations or challenges associated with using Tributyl(iodomethyl)stannane in organic synthesis?
A3: While Tributyl(iodomethyl)stannane is a valuable tool, its use presents certain challenges. The radical cyclization strategy, for instance, relies on controlling the reactivity of the imine intermediate, which can be unpredictable. [] Additionally, as with many organotin compounds, Tributyl(iodomethyl)stannane may present toxicity concerns, necessitating careful handling and disposal. []
Disclaimer and Information on In-Vitro Research Products
Please be aware that all articles and product information presented on BenchChem are intended solely for informational purposes. The products available for purchase on BenchChem are specifically designed for in-vitro studies, which are conducted outside of living organisms. In-vitro studies, derived from the Latin term "in glass," involve experiments performed in controlled laboratory settings using cells or tissues. It is important to note that these products are not categorized as medicines or drugs, and they have not received approval from the FDA for the prevention, treatment, or cure of any medical condition, ailment, or disease. We must emphasize that any form of bodily introduction of these products into humans or animals is strictly prohibited by law. It is essential to adhere to these guidelines to ensure compliance with legal and ethical standards in research and experimentation.