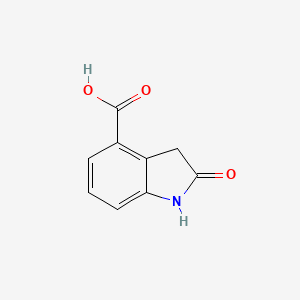
2-Oxoindoline-4-carboxylic acid
Overview
Description
2-Oxoindoline-4-carboxylic acid is a heterocyclic organic compound that belongs to the indole family It is characterized by a fused ring system consisting of a benzene ring and a pyrrole ring, with a carboxylic acid group at the fourth position and a ketone group at the second position
Mechanism of Action
Target of Action
The primary targets of 2-Oxoindoline-4-carboxylic acid are the RNA dependent RNA polymerase (RdRp) activity of DENV and procaspase-3 . These targets play a crucial role in viral replication and apoptosis respectively .
Mode of Action
This compound interacts with its targets by inhibiting the RdRp activity of DENV and activating procaspase-3 . This results in the inhibition of viral replication and induction of apoptosis .
Biochemical Pathways
The affected pathways include the viral replication pathway and the apoptotic pathway . The inhibition of RdRp activity disrupts the viral replication pathway, thereby preventing the spread of the virus . On the other hand, the activation of procaspase-3 triggers the apoptotic pathway, leading to cell death .
Pharmacokinetics
The compound’s notable cytotoxicity towards various human cancer cell lines suggests that it may have favorable pharmacokinetic properties .
Result of Action
The molecular and cellular effects of this compound’s action include the inhibition of viral replication and the induction of apoptosis . This leads to a decrease in the spread of the virus and the death of cancer cells .
Biochemical Analysis
Biochemical Properties
2-Oxoindoline-4-carboxylic acid plays a crucial role in several biochemical reactions. It interacts with various enzymes, proteins, and other biomolecules, influencing their activity and function. For instance, it has been shown to interact with enzymes involved in the metabolic pathways of amino acids and nucleotides. The compound can act as a substrate or inhibitor for these enzymes, thereby modulating their activity. Additionally, this compound can form complexes with proteins, affecting their structure and function. These interactions are essential for understanding the compound’s role in cellular metabolism and its potential therapeutic applications .
Cellular Effects
This compound has been observed to exert various effects on different types of cells and cellular processes. It influences cell function by modulating cell signaling pathways, gene expression, and cellular metabolism. For example, studies have shown that this compound can induce apoptosis in cancer cells by activating specific signaling pathways . It also affects the expression of genes involved in cell cycle regulation and apoptosis, leading to changes in cellular proliferation and survival. Furthermore, the compound can alter cellular metabolism by influencing the activity of key metabolic enzymes .
Molecular Mechanism
The molecular mechanism of action of this compound involves several key interactions at the molecular level. The compound can bind to specific biomolecules, such as enzymes and receptors, altering their activity. For instance, it has been shown to inhibit the activity of certain enzymes involved in nucleotide metabolism by binding to their active sites . This inhibition can lead to changes in the levels of nucleotides and other metabolites, affecting cellular processes. Additionally, this compound can modulate gene expression by interacting with transcription factors and other regulatory proteins .
Temporal Effects in Laboratory Settings
In laboratory settings, the effects of this compound can change over time due to its stability, degradation, and long-term impact on cellular function. The compound is relatively stable under standard laboratory conditions, but it can degrade over time, leading to changes in its biological activity . Long-term studies have shown that this compound can have sustained effects on cellular function, including prolonged inhibition of enzyme activity and persistent changes in gene expression . These temporal effects are important for understanding the compound’s potential therapeutic applications and its behavior in biological systems.
Dosage Effects in Animal Models
The effects of this compound vary with different dosages in animal models. At low doses, the compound can exert beneficial effects, such as inhibiting tumor growth and modulating immune responses . At high doses, it can cause toxic or adverse effects, including liver and kidney damage . These dosage-dependent effects are crucial for determining the therapeutic window and safety profile of this compound in preclinical studies.
Metabolic Pathways
This compound is involved in several metabolic pathways, including those related to amino acid and nucleotide metabolism. It interacts with enzymes such as aminotransferases and dehydrogenases, influencing the flux of metabolites through these pathways . The compound can also affect the levels of key metabolites, such as pyruvate and oxaloacetate, by modulating the activity of enzymes involved in their synthesis and degradation . These interactions are essential for understanding the compound’s role in cellular metabolism and its potential therapeutic applications.
Transport and Distribution
The transport and distribution of this compound within cells and tissues are mediated by specific transporters and binding proteins. The compound can be taken up by cells through active transport mechanisms, involving transporters such as organic anion transporters . Once inside the cell, this compound can bind to proteins and other biomolecules, influencing its localization and accumulation . These transport and distribution mechanisms are important for understanding the compound’s bioavailability and its effects on cellular function.
Subcellular Localization
The subcellular localization of this compound is influenced by its interactions with targeting signals and post-translational modifications. The compound can be directed to specific compartments or organelles within the cell, such as the mitochondria or nucleus, through interactions with targeting signals . These localization patterns can affect the compound’s activity and function, as well as its interactions with other biomolecules . Understanding the subcellular localization of this compound is crucial for elucidating its mechanism of action and potential therapeutic applications.
Preparation Methods
Synthetic Routes and Reaction Conditions: The synthesis of 2-Oxoindoline-4-carboxylic acid typically involves the cyclization of appropriate precursors. One common method is the reaction of isatin with malonic acid in the presence of a base, followed by decarboxylation. Another approach involves the use of indole-2-carboxylic acid derivatives, which undergo cyclization under acidic or basic conditions to form the desired product .
Industrial Production Methods: Industrial production of this compound may involve large-scale synthesis using optimized reaction conditions to ensure high yield and purity. The use of continuous flow reactors and automated synthesis platforms can enhance the efficiency and scalability of the production process .
Chemical Reactions Analysis
Types of Reactions: 2-Oxoindoline-4-carboxylic acid undergoes various chemical reactions, including:
Oxidation: The compound can be oxidized to form corresponding quinones or other oxidized derivatives.
Reduction: Reduction reactions can convert the ketone group to an alcohol, yielding 2-hydroxyindoline-4-carboxylic acid.
Common Reagents and Conditions:
Oxidation: Common oxidizing agents include potassium permanganate and chromium trioxide.
Reduction: Reducing agents such as sodium borohydride or lithium aluminum hydride are typically used.
Substitution: Electrophilic reagents like halogens or nitro groups can be introduced using appropriate catalysts and solvents.
Major Products Formed:
Oxidation: Formation of quinones or other oxidized derivatives.
Reduction: Formation of 2-hydroxyindoline-4-carboxylic acid.
Substitution: Formation of various substituted indoline derivatives.
Scientific Research Applications
2-Oxoindoline-4-carboxylic acid has a wide range of applications in scientific research:
Chemistry: It serves as a building block for the synthesis of complex organic molecules and natural products.
Biology: The compound is used in the study of enzyme inhibitors and as a probe for biological pathways.
Medicine: It has potential therapeutic applications, including anticancer and antimicrobial activities.
Industry: The compound is used in the development of dyes, pigments, and other industrial chemicals.
Comparison with Similar Compounds
- Indole-2-carboxylic acid
- Isatin
- 2-Hydroxyindoline-4-carboxylic acid
Comparison: 2-Oxoindoline-4-carboxylic acid is unique due to the presence of both a ketone and a carboxylic acid group, which imparts distinct chemical reactivity and biological activity. Compared to indole-2-carboxylic acid, it has an additional ketone group, enhancing its potential as a versatile synthetic intermediate. Isatin, another related compound, lacks the carboxylic acid group, resulting in different chemical properties and applications.
Properties
IUPAC Name |
2-oxo-1,3-dihydroindole-4-carboxylic acid | |
---|---|---|
Source | PubChem | |
URL | https://pubchem.ncbi.nlm.nih.gov | |
Description | Data deposited in or computed by PubChem | |
InChI |
InChI=1S/C9H7NO3/c11-8-4-6-5(9(12)13)2-1-3-7(6)10-8/h1-3H,4H2,(H,10,11)(H,12,13) | |
Source | PubChem | |
URL | https://pubchem.ncbi.nlm.nih.gov | |
Description | Data deposited in or computed by PubChem | |
InChI Key |
ALXJUSWINGKGAW-UHFFFAOYSA-N | |
Source | PubChem | |
URL | https://pubchem.ncbi.nlm.nih.gov | |
Description | Data deposited in or computed by PubChem | |
Canonical SMILES |
C1C2=C(C=CC=C2NC1=O)C(=O)O | |
Source | PubChem | |
URL | https://pubchem.ncbi.nlm.nih.gov | |
Description | Data deposited in or computed by PubChem | |
Molecular Formula |
C9H7NO3 | |
Source | PubChem | |
URL | https://pubchem.ncbi.nlm.nih.gov | |
Description | Data deposited in or computed by PubChem | |
DSSTOX Substance ID |
DTXSID60439190 | |
Record name | 2-Oxoindoline-4-carboxylic acid | |
Source | EPA DSSTox | |
URL | https://comptox.epa.gov/dashboard/DTXSID60439190 | |
Description | DSSTox provides a high quality public chemistry resource for supporting improved predictive toxicology. | |
Molecular Weight |
177.16 g/mol | |
Source | PubChem | |
URL | https://pubchem.ncbi.nlm.nih.gov | |
Description | Data deposited in or computed by PubChem | |
CAS No. |
90322-37-5 | |
Record name | 2-Oxoindoline-4-carboxylic acid | |
Source | EPA DSSTox | |
URL | https://comptox.epa.gov/dashboard/DTXSID60439190 | |
Description | DSSTox provides a high quality public chemistry resource for supporting improved predictive toxicology. | |
Synthesis routes and methods I
Procedure details
Synthesis routes and methods II
Procedure details
Retrosynthesis Analysis
AI-Powered Synthesis Planning: Our tool employs the Template_relevance Pistachio, Template_relevance Bkms_metabolic, Template_relevance Pistachio_ringbreaker, Template_relevance Reaxys, Template_relevance Reaxys_biocatalysis model, leveraging a vast database of chemical reactions to predict feasible synthetic routes.
One-Step Synthesis Focus: Specifically designed for one-step synthesis, it provides concise and direct routes for your target compounds, streamlining the synthesis process.
Accurate Predictions: Utilizing the extensive PISTACHIO, BKMS_METABOLIC, PISTACHIO_RINGBREAKER, REAXYS, REAXYS_BIOCATALYSIS database, our tool offers high-accuracy predictions, reflecting the latest in chemical research and data.
Strategy Settings
Precursor scoring | Relevance Heuristic |
---|---|
Min. plausibility | 0.01 |
Model | Template_relevance |
Template Set | Pistachio/Bkms_metabolic/Pistachio_ringbreaker/Reaxys/Reaxys_biocatalysis |
Top-N result to add to graph | 6 |
Feasible Synthetic Routes
Disclaimer and Information on In-Vitro Research Products
Please be aware that all articles and product information presented on BenchChem are intended solely for informational purposes. The products available for purchase on BenchChem are specifically designed for in-vitro studies, which are conducted outside of living organisms. In-vitro studies, derived from the Latin term "in glass," involve experiments performed in controlled laboratory settings using cells or tissues. It is important to note that these products are not categorized as medicines or drugs, and they have not received approval from the FDA for the prevention, treatment, or cure of any medical condition, ailment, or disease. We must emphasize that any form of bodily introduction of these products into humans or animals is strictly prohibited by law. It is essential to adhere to these guidelines to ensure compliance with legal and ethical standards in research and experimentation.