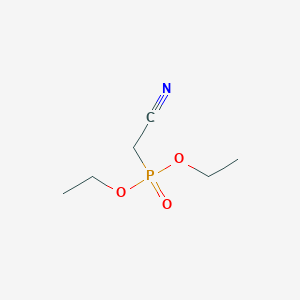
Diethyl cyanomethylphosphonate
Overview
Description
Diethyl cyanomethylphosphonate is an organophosphorus compound with the molecular formula C6H12NO3P. It is a clear, colorless to pale yellow liquid that is miscible with water, chloroform, tetrahydrofuran, ethyl acetate, and methylene chloride . This compound is primarily used as an intermediate in various chemical reactions, including the Horner-Emmons reaction, which is a modification of the Wittig reaction used to synthesize substituted nitriles .
Mechanism of Action
Target of Action
Diethyl cyanomethylphosphonate is a biochemical reagent . It is primarily used as a reagent in the Horner-Wadsworth-Emmons (HWE) olefination reaction . The primary targets of this compound are aldehydes and ketones, which it helps to convert into α,β-unsaturated nitriles .
Mode of Action
The compound acts by participating in the HWE olefination reaction . This reaction involves the conversion of carbonyl compounds (aldehydes and ketones) into α,β-unsaturated nitriles . The cyanomethylphosphonate group in the compound plays a crucial role in this transformation .
Biochemical Pathways
The HWE olefination reaction is a key biochemical pathway affected by this compound . This reaction is a method for forming carbon-carbon double bonds (olefins) and is widely used in organic synthesis . The downstream effects include the production of α,β-unsaturated nitriles, which are important intermediates in various chemical syntheses .
Result of Action
The primary result of the action of this compound is the conversion of aldehydes and ketones into α,β-unsaturated nitriles . This transformation is crucial in the synthesis of various organic compounds .
Action Environment
The action of this compound can be influenced by various environmental factors. For instance, the efficiency of the HWE olefination reaction can be affected by the reaction temperature, the solvent used, and the presence of other reactants . Additionally, the stability of the compound may be influenced by storage conditions .
Biochemical Analysis
. .
Biochemical Properties
Diethyl cyanomethylphosphonate plays a role in biochemical reactions, particularly in the synthesis of substituted nitriles and their amide and heterocyclic derivatives .
Cellular Effects
While specific cellular effects of this compound are not widely documented, it has been suggested that it has anti-tumor and antibacterial biological activities . This is due to this compound’s role in biochemical reactions where it acts as an aminotransferase .
Molecular Mechanism
This compound reacts with epoxides and nitrones to prepare cyano-substituted cyclopropanes and aziridines respectively . It is actively involved in the synthesis of alpha-arylated alkanenitriles via reaction with aryl iodides in the presence of CuI .
Preparation Methods
Synthetic Routes and Reaction Conditions: The preparation of diethyl cyanomethylphosphonate typically involves the following steps:
Synthesis of Chloromethylphosphonic Acid Diethyl Ester:
Conversion to this compound:
Industrial Production Methods: Industrial production of this compound follows similar synthetic routes but on a larger scale. The reaction conditions are optimized to ensure high yield and purity. The process involves:
Refluxing Chloromethylphosphonic Dichloride with Ethanol:
Reaction with Sodium Cyanide:
Chemical Reactions Analysis
Types of Reactions: Diethyl cyanomethylphosphonate undergoes various chemical reactions, including:
Substitution Reactions:
Horner-Emmons Reaction:
Common Reagents and Conditions:
Epoxides and Nitrones:
Aryl Iodides and Copper(I) Iodide:
Major Products:
Cyano-Substituted Cyclopropanes and Aziridines:
Alpha, Beta-Unsaturated Nitriles:
Scientific Research Applications
Diethyl cyanomethylphosphonate has several applications in scientific research:
Biology and Medicine:
Comparison with Similar Compounds
Biological Activity
Diethyl cyanomethylphosphonate (DCMP), also known as diethyl (cyanomethyl)phosphonate, is a chemical compound with significant biological activity and applications in various fields, including medicinal chemistry and agrochemicals. This article provides a detailed overview of the biological activity of DCMP, including its chemical properties, mechanisms of action, and relevant research findings.
- Molecular Formula : CHNOP
- Molecular Weight : 177.14 g/mol
- Density : 1.1 g/cm³
- Boiling Point : 305.2 °C
- Flash Point : 117.3 °C
DCMP is characterized by its phosphonate structure, which plays a crucial role in its biological interactions. The compound is often utilized as a biochemical reagent in life sciences research due to its reactivity and ability to form stable complexes with biomolecules.
Mechanisms of Biological Activity
This compound exhibits several mechanisms of action that contribute to its biological activity:
- Nerve Agent Simulant : DCMP is recognized as a simulant for nerve agents like Tabun. It can interact with acetylcholinesterase, an enzyme critical for nerve function, thereby mimicking the effects of toxic nerve agents without the associated lethality .
- DNA Binding : Research indicates that DCMP can form complexes with DNA through electrostatic interactions and minor-groove binding. This property is particularly relevant in the design of anticancer agents .
- Catalytic Activity : DCMP has been employed in various catalytic processes, including micellar catalysis, where it facilitates reactions involving hydrogen ions . Its ability to stabilize reactive intermediates enhances its utility in synthetic organic chemistry.
Study 1: Interaction with Acetylcholinesterase
A study investigated the interaction of DCMP with acetylcholinesterase, demonstrating that it inhibits the enzyme's activity in a concentration-dependent manner. This inhibition was reversible and indicated potential for developing therapeutic agents targeting cholinergic systems .
Study 2: Synthesis of Phosphonate Derivatives
In another research effort, DCMP was utilized to synthesize various phosphonate derivatives through nucleophilic substitution reactions. These derivatives exhibited enhanced biological activity against certain cancer cell lines, suggesting that modifications to the phosphonate group can lead to improved therapeutic profiles .
Study 3: DNA Complex Formation
A significant study focused on the ability of DCMP to bind DNA selectively. The results showed that DCMP could effectively intercalate into DNA strands, leading to structural alterations that may inhibit replication processes in cancer cells .
Data Table: Biological Activity Overview
Properties
IUPAC Name |
2-diethoxyphosphorylacetonitrile | |
---|---|---|
Source | PubChem | |
URL | https://pubchem.ncbi.nlm.nih.gov | |
Description | Data deposited in or computed by PubChem | |
InChI |
InChI=1S/C6H12NO3P/c1-3-9-11(8,6-5-7)10-4-2/h3-4,6H2,1-2H3 | |
Source | PubChem | |
URL | https://pubchem.ncbi.nlm.nih.gov | |
Description | Data deposited in or computed by PubChem | |
InChI Key |
KWMBADTWRIGGGG-UHFFFAOYSA-N | |
Source | PubChem | |
URL | https://pubchem.ncbi.nlm.nih.gov | |
Description | Data deposited in or computed by PubChem | |
Canonical SMILES |
CCOP(=O)(CC#N)OCC | |
Source | PubChem | |
URL | https://pubchem.ncbi.nlm.nih.gov | |
Description | Data deposited in or computed by PubChem | |
Molecular Formula |
C6H12NO3P | |
Source | PubChem | |
URL | https://pubchem.ncbi.nlm.nih.gov | |
Description | Data deposited in or computed by PubChem | |
DSSTOX Substance ID |
DTXSID20180015 | |
Record name | Diethyl (cyanomethyl)phosphonate | |
Source | EPA DSSTox | |
URL | https://comptox.epa.gov/dashboard/DTXSID20180015 | |
Description | DSSTox provides a high quality public chemistry resource for supporting improved predictive toxicology. | |
Molecular Weight |
177.14 g/mol | |
Source | PubChem | |
URL | https://pubchem.ncbi.nlm.nih.gov | |
Description | Data deposited in or computed by PubChem | |
CAS No. |
2537-48-6 | |
Record name | Diethyl P-(cyanomethyl)phosphonate | |
Source | CAS Common Chemistry | |
URL | https://commonchemistry.cas.org/detail?cas_rn=2537-48-6 | |
Description | CAS Common Chemistry is an open community resource for accessing chemical information. Nearly 500,000 chemical substances from CAS REGISTRY cover areas of community interest, including common and frequently regulated chemicals, and those relevant to high school and undergraduate chemistry classes. This chemical information, curated by our expert scientists, is provided in alignment with our mission as a division of the American Chemical Society. | |
Explanation | The data from CAS Common Chemistry is provided under a CC-BY-NC 4.0 license, unless otherwise stated. | |
Record name | Diethyl (cyanomethyl)phosphonate | |
Source | ChemIDplus | |
URL | https://pubchem.ncbi.nlm.nih.gov/substance/?source=chemidplus&sourceid=0002537486 | |
Description | ChemIDplus is a free, web search system that provides access to the structure and nomenclature authority files used for the identification of chemical substances cited in National Library of Medicine (NLM) databases, including the TOXNET system. | |
Record name | Diethyl (cyanomethyl)phosphonate | |
Source | DTP/NCI | |
URL | https://dtp.cancer.gov/dtpstandard/servlet/dwindex?searchtype=NSC&outputformat=html&searchlist=407826 | |
Description | The NCI Development Therapeutics Program (DTP) provides services and resources to the academic and private-sector research communities worldwide to facilitate the discovery and development of new cancer therapeutic agents. | |
Explanation | Unless otherwise indicated, all text within NCI products is free of copyright and may be reused without our permission. Credit the National Cancer Institute as the source. | |
Record name | Diethyl (cyanomethyl)phosphonate | |
Source | EPA DSSTox | |
URL | https://comptox.epa.gov/dashboard/DTXSID20180015 | |
Description | DSSTox provides a high quality public chemistry resource for supporting improved predictive toxicology. | |
Record name | Diethyl (cyanomethyl)phosphonate | |
Source | European Chemicals Agency (ECHA) | |
URL | https://echa.europa.eu/substance-information/-/substanceinfo/100.018.006 | |
Description | The European Chemicals Agency (ECHA) is an agency of the European Union which is the driving force among regulatory authorities in implementing the EU's groundbreaking chemicals legislation for the benefit of human health and the environment as well as for innovation and competitiveness. | |
Explanation | Use of the information, documents and data from the ECHA website is subject to the terms and conditions of this Legal Notice, and subject to other binding limitations provided for under applicable law, the information, documents and data made available on the ECHA website may be reproduced, distributed and/or used, totally or in part, for non-commercial purposes provided that ECHA is acknowledged as the source: "Source: European Chemicals Agency, http://echa.europa.eu/". Such acknowledgement must be included in each copy of the material. ECHA permits and encourages organisations and individuals to create links to the ECHA website under the following cumulative conditions: Links can only be made to webpages that provide a link to the Legal Notice page. | |
Retrosynthesis Analysis
AI-Powered Synthesis Planning: Our tool employs the Template_relevance Pistachio, Template_relevance Bkms_metabolic, Template_relevance Pistachio_ringbreaker, Template_relevance Reaxys, Template_relevance Reaxys_biocatalysis model, leveraging a vast database of chemical reactions to predict feasible synthetic routes.
One-Step Synthesis Focus: Specifically designed for one-step synthesis, it provides concise and direct routes for your target compounds, streamlining the synthesis process.
Accurate Predictions: Utilizing the extensive PISTACHIO, BKMS_METABOLIC, PISTACHIO_RINGBREAKER, REAXYS, REAXYS_BIOCATALYSIS database, our tool offers high-accuracy predictions, reflecting the latest in chemical research and data.
Strategy Settings
Precursor scoring | Relevance Heuristic |
---|---|
Min. plausibility | 0.01 |
Model | Template_relevance |
Template Set | Pistachio/Bkms_metabolic/Pistachio_ringbreaker/Reaxys/Reaxys_biocatalysis |
Top-N result to add to graph | 6 |
Feasible Synthetic Routes
Q1: What is the structural formula, molecular weight, and key spectroscopic data for diethyl cyanomethylphosphonate?
A1: this compound has the molecular formula C6H12NO3P and a molecular weight of 177.13 g/mol. While the provided research excerpts do not include detailed spectroscopic data, key characteristics can be deduced. In 1H NMR, we expect signals for the ethyl groups (triplet and quartet) and the methylene group adjacent to the phosphonate (doublet). 13C NMR would reveal signals for all carbons, with the cyano carbon exhibiting a characteristic shift. IR spectroscopy would show a strong band for the nitrile (C≡N) stretch.
Q2: How does this compound typically react with carbonyl compounds?
A2: this compound readily undergoes Horner–Wadsworth–Emmons (HWE) olefination reactions with aldehydes and ketones. This reaction, often conducted in the presence of a base, forms α,β-unsaturated nitriles with high stereoselectivity. [, ]
Q3: Can this compound participate in reactions other than HWE olefinations?
A3: Yes, research indicates its involvement in other reactions. For example, it participates in Knoevenagel condensations with aldehydes, yielding α,β-unsaturated nitriles, sometimes in competition with the HWE product. [, , ] Additionally, it reacts with epoxides, leading to ring-opening and the formation of cyclopropylphosphonate derivatives. []
Q4: How is this compound utilized in the synthesis of biologically active compounds?
A4: this compound serves as a key building block for synthesizing various biologically relevant molecules. Its use in constructing branched-chain sugar nucleosides, potential HIV inhibitors, highlights its versatility. [] It also facilitates the synthesis of phthalocyanines with potential applications in photodynamic therapy due to their unique photophysical properties. []
Q5: Can you elaborate on the use of this compound in the synthesis of organophosphorus group substituted fullerenes?
A5: Research demonstrates the reaction of this compound with fullerenes (C60 and C70) under basic conditions, resulting in organophosphorus functionalized fullerene derivatives. These derivatives exhibit potential in materials science due to the unique electronic properties imparted by the phosphonate group. []
Q6: What about its role in preparing porous metal phosphonate materials?
A6: this compound acts as a precursor for synthesizing porous metal phosphonates. Under hydrothermal conditions, it reacts with metal salts, undergoing hydrolysis to generate phosphonate anions. These anions, along with the metal cations, assemble into frameworks containing channels, offering potential applications in catalysis and separation processes. [, ]
Q7: Does the stereochemistry of the double bond formed during the HWE reaction with this compound vary?
A7: Yes, the stereochemical outcome of the HWE reaction involving this compound can be influenced by various factors, including the reaction conditions and the nature of the carbonyl compound. Research highlights instances where specific reaction conditions favor either the (E)- or (Z)-isomer of the resulting α,β-unsaturated nitrile. [, ]
Q8: Are there studies exploring the structure-activity relationships of compounds derived from this compound?
A8: Yes, researchers have investigated the impact of structural modifications on the biological activity of this compound derivatives. Studies on branched-chain sugar nucleosides, for instance, reveal the influence of stereochemistry and protecting groups on their activity as potential HIV inhibitors. [] Similarly, in the context of anticancer agents, research highlights the significant impact of various nitrogen- and carbon-centered substituents introduced via Michael addition on the cytotoxicity profiles of arglabin derivatives. []
Disclaimer and Information on In-Vitro Research Products
Please be aware that all articles and product information presented on BenchChem are intended solely for informational purposes. The products available for purchase on BenchChem are specifically designed for in-vitro studies, which are conducted outside of living organisms. In-vitro studies, derived from the Latin term "in glass," involve experiments performed in controlled laboratory settings using cells or tissues. It is important to note that these products are not categorized as medicines or drugs, and they have not received approval from the FDA for the prevention, treatment, or cure of any medical condition, ailment, or disease. We must emphasize that any form of bodily introduction of these products into humans or animals is strictly prohibited by law. It is essential to adhere to these guidelines to ensure compliance with legal and ethical standards in research and experimentation.