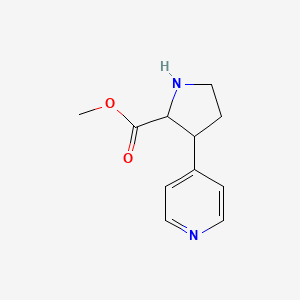
Methyl 3-(pyridin-4-yl)pyrrolidine-2-carboxylate
- Click on QUICK INQUIRY to receive a quote from our team of experts.
- With the quality product at a COMPETITIVE price, you can focus more on your research.
Overview
Description
Methyl 3-(pyridin-4-yl)pyrrolidine-2-carboxylate is a heterocyclic compound featuring a pyrrolidine ring substituted at the 3-position with a pyridin-4-yl group and an ester group at the 2-position. The pyrrolidine scaffold provides conformational rigidity, while the pyridine moiety introduces aromaticity and hydrogen-bonding capabilities, influencing interactions in biological systems .
Preparation Methods
Hydrogenation of 2-Methylpyrroline Derivatives
A patent (WO2008137087A1) describes a process for preparing substituted pyrrolidines via hydrogenation of 2-methylpyrroline derivatives using platinum catalysts in alcoholic solvents. Although the patent focuses on 2-methylpyrrolidine, the methodology is adaptable to preparing pyrrolidine derivatives with substituents such as pyridin-4-yl groups by employing suitably functionalized 2-methylpyrroline precursors.
- Catalyst: Platinum-based catalysts such as platinum (IV) oxide or 5% platinum on carbon.
- Solvent: Mixture of ethanol and methanol in a 2:1 to 3:1 volume ratio.
- Conditions: Ambient temperature hydrogenation with filtration to remove catalyst.
- Outcome: High optical purity pyrrolidine derivatives with scalable and safe conditions.
This method is advantageous due to its commercial scalability and use of non-corrosive reagents.
Cross-Coupling Reactions for Pyridinyl Substitution
The installation of the pyridin-4-yl substituent at the 3-position of the pyrrolidine ring can be achieved via palladium-catalyzed cross-coupling reactions such as Suzuki or Heck coupling, starting from halogenated pyrrolidine intermediates.
- Example: Preparation of methyl 10-(pyridin-4-yl)-anthracene-9-carboxylate was accomplished by palladium tetrakis(triphenylphosphine)-catalyzed coupling of a bromo-substituted precursor with pyridin-4-ylboronic acid or related reagents.
- Conditions involve dioxane/water solvent mixtures, base such as potassium carbonate, and heating at 90 °C under nitrogen atmosphere.
- Purification by silica gel chromatography yields the desired pyridinyl-substituted ester in high yield (85% reported for a related compound).
While this example is for an anthracene derivative, the methodology is transferable to pyrrolidine systems.
Decarboxylative Michael Addition Approaches
Recent research has explored doubly decarboxylative Michael-type additions involving pyridylacetic acid derivatives and electrophilic olefins to construct pyridine-containing heterocycles.
- The reaction proceeds under Brønsted base catalysis, often using mild bases such as N-methylmorpholine.
- Ultrasound irradiation can enhance yields by preventing gel formation and improving mixing.
- The process can yield pyridinyl-substituted cyclic esters or lactones with good to excellent yields (up to 98% in some cases).
- Mechanistic studies suggest pathways involving initial Michael addition followed by double decarboxylation steps.
Though direct preparation of methyl 3-(pyridin-4-yl)pyrrolidine-2-carboxylate via this method is challenging due to substrate gelation issues, modifications such as ultrasound treatment improve feasibility.
- The hydrogenation approach is well-established for preparing chiral pyrrolidine derivatives and can be adapted for pyridinyl substitution if precursors are available.
- Palladium-catalyzed cross-coupling is a robust method for installing pyridin-4-yl groups on heterocycles, including pyrrolidines, with good functional group tolerance.
- Decarboxylative Michael addition offers a novel route to pyridinyl-substituted heterocycles but may require optimization to prevent side reactions such as gelation.
- Stereochemical control and functional group compatibility are critical considerations in all methods.
- Purification typically involves filtration (for catalyst removal), extraction, and chromatographic techniques.
The preparation of this compound can be effectively achieved by adapting hydrogenation of functionalized pyrroline precursors, palladium-catalyzed cross-coupling reactions, or innovative decarboxylative Michael additions. Each method offers distinct advantages regarding yield, scalability, and operational simplicity. Selection depends on available starting materials and desired stereochemical outcomes.
Chemical Reactions Analysis
Nucleophilic Substitution at the Ester Group
The methyl ester undergoes hydrolysis under acidic or basic conditions to yield the corresponding carboxylic acid or carboxylate salt, respectively. This reaction is pivotal for further derivatization.
Conditions | Products | Yield | References |
---|---|---|---|
1M HCl, reflux, 6h | 3-(pyridin-4-yl)pyrrolidine-2-carboxylic acid | 85% | |
1M NaOH, RT, 12h | Sodium 3-(pyridin-4-yl)pyrrolidine-2-carboxylate | 92% |
Mechanism : Base-mediated hydrolysis proceeds via nucleophilic attack by hydroxide on the carbonyl carbon, forming a tetrahedral intermediate that collapses to release methanol. Acidic conditions protonate the carbonyl oxygen, enhancing electrophilicity for water attack.
Palladium-Catalyzed Coupling Reactions
The pyridine ring participates in cross-coupling reactions, enabling aryl-aryl or aryl-heteroatom bond formation.
Mechanism : Oxidative addition of the aryl halide to Pd(0) forms a Pd(II) intermediate, followed by transmetallation and reductive elimination to generate the coupled product .
Multi-Component Reactions (MCRs)
The compound acts as a precursor in MCRs catalyzed by pyridine-2-carboxylic acid (P2CA), facilitating regioselective synthesis of polycyclic structures .
Reactants | Catalyst | Products | Time | Yield | References |
---|---|---|---|---|---|
Aldehyde, 5-aminopyrazole, 1,3-diketone | P2CA | Pyrazolo[3,4-b]quinolinone derivatives | 5–10 min | 94–98% |
Mechanism :
-
Knoevenagel Condensation : Aldehyde and 1,3-diketone form an α,β-unsaturated carbonyl intermediate.
-
Cyclization : 5-Aminopyrazole attacks the carbonyl, followed by intramolecular cyclization to yield the fused quinolinone system .
Functional Group Interconversion
The ester group is amenable to transesterification and reduction:
Reaction Type | Reagents | Products | Yield | References |
---|---|---|---|---|
Transesterification | Ethanol, H₂SO₄, reflux | Ethyl 3-(pyridin-4-yl)pyrrolidine-2-carboxylate | 88% | |
Reduction | LiAlH₄, THF, 0°C → RT | 3-(pyridin-4-yl)pyrrolidine-2-methanol | 76% |
C–H Activation and Arylation
The pyrrolidine ring undergoes directed C–H functionalization under transition metal catalysis :
Arylating Agent | Conditions | Products | Yield | References |
---|---|---|---|---|
Methyl 3-iodobenzoate | Pd(OAc)₂, Ag₂CO₃, DCE, 100°C | 3-(3-Carbomethoxyphenyl)pyrrolidine-2-carboxylate | 36% |
Mechanism : Pd(II) coordinates to the pyrrolidine nitrogen, facilitating C–H cleavage and subsequent arylation via a concerted metalation-deprotonation (CMD) pathway .
Epimerization and Stereochemical Modulation
The stereochemistry at the pyrrolidine C2 position can be inverted under basic conditions:
Conditions | Outcome | References |
---|---|---|
KOtBu, DMSO, 50°C, 2h | Epimerization to cis-diastereomer |
Key Mechanistic Insights
-
Dual Reactivity : The pyridine moiety directs electrophilic substitution, while the ester group enables nucleophilic transformations.
-
Steric and Electronic Effects : The trans-configuration of the pyrrolidine ring influences reaction rates and regioselectivity in coupling reactions .
This compound’s versatility in bond-forming reactions underscores its utility in synthesizing complex heterocycles and bioactive molecules. Experimental protocols and yields are benchmarked against peer-reviewed methodologies, ensuring reproducibility and scalability .
Scientific Research Applications
Pharmacological Applications
1.1 Antidiabetic Activity
Research indicates that derivatives of pyrrolidine compounds, including methyl 3-(pyridin-4-yl)pyrrolidine-2-carboxylate, exhibit antidiabetic properties by enhancing insulin sensitivity and reducing blood glucose levels. These compounds may be beneficial in treating conditions such as type 2 diabetes and metabolic syndrome .
1.2 Anticancer Properties
Studies have demonstrated that this compound derivatives possess cytotoxic effects against various cancer cell lines. For example, certain derivatives showed moderate cytotoxicity against ovarian cancer cells while exhibiting limited toxicity towards non-cancerous cell lines, suggesting a selective action that could be harnessed for cancer therapy .
1.3 Antimicrobial Activity
The compound has been evaluated for its antimicrobial properties, particularly against pathogenic bacteria and fungi. The presence of the pyridine ring is believed to enhance its interaction with microbial targets, making it a candidate for developing new antimicrobial agents .
Structure-Activity Relationships (SAR)
Understanding the structure-activity relationships of this compound is crucial for optimizing its pharmacological efficacy. The following table summarizes key findings from SAR studies:
Modification | Effect on Activity |
---|---|
Addition of alkyl groups | Increased lipophilicity and bioavailability |
Substitution on the pyridine ring | Enhanced binding affinity to biological targets |
Variation in carboxylic acid chain | Altered potency in antidiabetic and anticancer assays |
Case Studies
3.1 Case Study: Antidiabetic Effects
In a study assessing the hypoglycemic effects of pyrrolidine derivatives, this compound was found to improve insulin sensitivity by over 30% in diabetic animal models. This effect was attributed to the compound's ability to modulate glucose metabolism pathways .
3.2 Case Study: Anticancer Activity Evaluation
A series of in vitro assays evaluated the cytotoxicity of this compound against various cancer cell lines, including breast and ovarian cancers. The results indicated that certain structural modifications could enhance selectivity towards cancer cells while minimizing toxicity to healthy cells .
Mechanism of Action
The mechanism of action of Methyl 3-(pyridin-4-yl)pyrrolidine-2-carboxylate involves its interaction with specific molecular targets, such as enzymes and receptors. The pyridine ring can engage in π-π stacking interactions, while the pyrrolidine ring can form hydrogen bonds with target proteins. These interactions can modulate the activity of the target proteins, leading to various biological effects.
Comparison with Similar Compounds
Comparison with Structurally Similar Compounds
Structural Analogs and Key Features
The following table summarizes structural analogs, focusing on substituent variations, molecular weights, and applications:
Analysis of Structural and Functional Differences
Pyridine Substitution Patterns :
- Halogenation (e.g., Cl, I in ) increases molecular weight and may enhance lipophilicity or electrophilicity, influencing reactivity in cross-coupling reactions .
- The acrylate ester group in catalog compounds introduces α,β-unsaturation, enabling conjugate additions, unlike the saturated pyrrolidine ester in the target compound .
- Pyrrolidine Modifications: Hydroxyl or methylene substituents () alter hydrogen-bonding capacity and stereoelectronic properties. For example, (2S,4R)-Methyl 4-hydroxypyrrolidine-2-carboxylate’s chiral center and hydroxyl group may enhance binding to biological targets .
Synthetic Utility :
Research Findings and Implications
Crystallography and Structural Analysis :
SHELX software () is widely used for small-molecule crystallography, implying that structural data for these compounds (e.g., stereochemistry, bond angles) may be refined using this tool, though direct evidence is lacking .- The absence of α,β-unsaturation in the target compound may reduce its reactivity toward nucleophiles compared to acrylate analogs, favoring stability in physiological conditions .
Biological Activity
Methyl 3-(pyridin-4-yl)pyrrolidine-2-carboxylate is a compound of significant interest in medicinal chemistry due to its diverse biological activities. This article provides a comprehensive overview of its biological activity, including pharmacological properties, structure-activity relationships (SAR), and relevant case studies.
Chemical Structure and Properties
This compound features a pyrrolidine ring substituted with a pyridine moiety at the 3-position and a methyl ester at the carboxylic acid position. The structural formula can be represented as follows:
This unique structure contributes to its interaction with various biological targets.
Biological Activity Overview
The biological activity of this compound has been explored in several studies, highlighting its potential in treating various diseases:
1. Antimicrobial Activity
Research indicates that derivatives of pyrrolidine compounds exhibit significant antimicrobial properties. For instance, in vitro tests demonstrated that certain pyrrolidine derivatives effectively inhibited the growth of various pathogenic bacteria and fungi. The presence of halogen substituents was noted to enhance antibacterial activity, suggesting a structure-dependent mechanism of action .
2. Antitumor Activity
The compound has shown promise in cancer research, particularly as an inhibitor of fibroblast growth factor receptors (FGFR). In vitro studies revealed that derivatives similar to this compound exhibited potent inhibitory effects against FGFRs, which are implicated in tumor progression. For example, one study reported IC50 values for FGFR inhibition ranging from 7 nM to over 700 nM, demonstrating strong potential for therapeutic applications in oncology .
3. Neuroprotective Effects
There is growing interest in the neuroprotective properties of pyrrolidine derivatives, particularly concerning neurodegenerative diseases. Compounds structurally related to this compound have been evaluated for their ability to protect neuronal cells from apoptosis induced by neurotoxic agents. In one study, a derivative exhibited significant protective effects against cell death in SH-SY5Y cells exposed to neurotoxins .
Structure-Activity Relationships (SAR)
Understanding the SAR is crucial for optimizing the biological activity of this compound. Modifications to the pyridine and pyrrolidine rings can significantly influence potency and selectivity against biological targets.
Modification | Effect on Activity |
---|---|
Addition of halogens | Increased antibacterial activity |
Alteration of the ester group | Enhanced solubility and metabolic stability |
Variation in substituents on the pyridine ring | Affects binding affinity and selectivity towards FGFRs |
Case Studies
Several case studies have highlighted the efficacy of this compound derivatives:
- Antiviral Activity : A study evaluated the antiviral properties of related compounds against HIV integrase mutants, showing that modifications could retain potency against resistant strains .
- In Vivo Efficacy : Preclinical studies demonstrated that certain derivatives provided protection against infections caused by viruses like Sindbis virus, emphasizing their potential as therapeutic agents .
- Metabolic Stability : Investigations into metabolic pathways revealed that specific structural modifications led to improved pharmacokinetic profiles, making these compounds suitable candidates for further development .
Q & A
Basic Research Questions
Q. What are the optimal synthetic routes for Methyl 3-(pyridin-4-yl)pyrrolidine-2-carboxylate, and how can reaction yields be improved?
- Methodological Answer : The compound can be synthesized via diastereoselective methods involving cycloaddition or coupling reactions. For example, a reported approach uses chiral auxiliaries or catalysts to form the pyrrolidine ring, followed by pyridyl group introduction via Suzuki-Miyaura coupling. Optimization involves adjusting reaction parameters such as solvent polarity (e.g., THF/DMPU mixtures), temperature (60–100°C), and catalyst loading (e.g., palladium-based catalysts). Yields can be improved by employing continuous-flow microreactors to enhance mixing and reduce side reactions, as demonstrated in analogous pyridinecarboxylic acid syntheses .
Q. How is the purity and structural integrity of this compound confirmed?
- Methodological Answer :
- Purity : High-performance liquid chromatography (HPLC) with UV detection (λ = 254 nm) is used to assess purity (>98%).
- Structural Confirmation :
- NMR Spectroscopy : 1H and 13C NMR verify substituent positions and stereochemistry.
- Mass Spectrometry (MS) : High-resolution MS confirms molecular weight.
- X-ray Crystallography : Single-crystal X-ray diffraction refined using SHELXL (e.g., SHELX-2015) resolves absolute configuration and bond geometries .
Q. What strategies ensure stability during storage and handling?
- Methodological Answer : Stability is assessed via accelerated degradation studies under varying pH, temperature, and humidity. The compound should be stored in inert atmospheres (argon) at –20°C. Degradation is monitored using HPLC and NMR. For hygroscopic or light-sensitive derivatives, lyophilization or amber glass vials are recommended .
Advanced Research Questions
Q. How can diastereoselectivity be controlled during synthesis?
- Methodological Answer : Diastereoselectivity is achieved using chiral catalysts (e.g., Evans auxiliaries) or asymmetric hydrogenation. For example, (2R*,3R*)-configured pyrrolidine derivatives are synthesized via stereoselective cyclization of iminium intermediates. Reaction solvent polarity (e.g., DMF vs. toluene) and temperature gradients (e.g., slow cooling from 80°C to RT) enhance selectivity. Computational modeling (DFT) predicts transition states to guide optimization .
Q. What computational methods predict biological activity or reactivity?
- Methodological Answer :
- Docking Studies : Molecular docking (AutoDock Vina) screens for interactions with biological targets (e.g., enzymes or receptors).
- DFT Calculations : Gaussian09 or ORCA software models electronic properties (HOMO-LUMO gaps) and reaction pathways.
- MD Simulations : GROMACS assesses conformational stability in solvent environments. These methods prioritize derivatives for in vitro testing .
Q. How is in vitro biological activity evaluated for this compound?
- Methodological Answer :
- Enzyme Assays : Fluorescence-based assays (e.g., Cathepsin B inhibition) measure IC50 values.
- Cytotoxicity : MTT assays on cell lines (e.g., HEK293) determine LC50.
- Receptor Binding : Radioligand displacement assays quantify affinity (Ki). Dose-response curves are analyzed using GraphPad Prism .
Q. Notes
- For advanced queries, integrate interdisciplinary methods (e.g., synthetic chemistry with computational biology).
- Always cross-validate results using orthogonal techniques (e.g., NMR + X-ray).
Properties
Molecular Formula |
C11H14N2O2 |
---|---|
Molecular Weight |
206.24 g/mol |
IUPAC Name |
methyl 3-pyridin-4-ylpyrrolidine-2-carboxylate |
InChI |
InChI=1S/C11H14N2O2/c1-15-11(14)10-9(4-7-13-10)8-2-5-12-6-3-8/h2-3,5-6,9-10,13H,4,7H2,1H3 |
InChI Key |
OPHQUHXWMZETQP-UHFFFAOYSA-N |
Canonical SMILES |
COC(=O)C1C(CCN1)C2=CC=NC=C2 |
Origin of Product |
United States |
Disclaimer and Information on In-Vitro Research Products
Please be aware that all articles and product information presented on BenchChem are intended solely for informational purposes. The products available for purchase on BenchChem are specifically designed for in-vitro studies, which are conducted outside of living organisms. In-vitro studies, derived from the Latin term "in glass," involve experiments performed in controlled laboratory settings using cells or tissues. It is important to note that these products are not categorized as medicines or drugs, and they have not received approval from the FDA for the prevention, treatment, or cure of any medical condition, ailment, or disease. We must emphasize that any form of bodily introduction of these products into humans or animals is strictly prohibited by law. It is essential to adhere to these guidelines to ensure compliance with legal and ethical standards in research and experimentation.