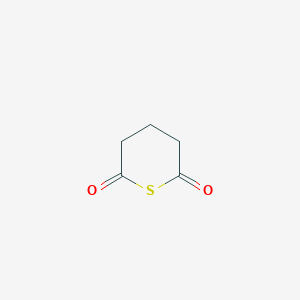
Glutaric thioanhydride
- Click on QUICK INQUIRY to receive a quote from our team of experts.
- With the quality product at a COMPETITIVE price, you can focus more on your research.
Overview
Description
Glutaric thioanhydride (GTA), a sulfur-containing analog of glutaric anhydride, is characterized by the replacement of oxygen atoms in the anhydride group with sulfur. This structural modification imparts distinct chemical reactivity and biological properties. GTA is primarily utilized in polymerization processes, such as ring-opening copolymerization (ROCOP) with epoxides or thiolanes, catalyzed by Lewis bases or PPNX systems, to produce functional polymers with antimicrobial properties . Its applications span pharmaceuticals, agrochemicals, and materials science, driven by its unique electronic and steric properties compared to oxygen-based analogs.
Preparation Methods
Synthetic Routes and Reaction Conditions
Dihydro-2H-thiopyran-2,6(3H)-dione can be synthesized through several methods. One common approach involves the cyclization of 1,4-dithiols with appropriate carbonyl compounds under acidic conditions. The reaction typically proceeds via the formation of a thioacetal intermediate, which then undergoes cyclization to form the thiopyran ring.
Industrial Production Methods
In an industrial setting, the production of dihydro-2H-thiopyran-2,6(3H)-dione may involve the use of continuous flow reactors to ensure efficient mixing and reaction control. The use of catalysts, such as Lewis acids, can enhance the reaction rate and yield. Additionally, purification steps, including distillation and recrystallization, are employed to obtain the desired product with high purity.
Chemical Reactions Analysis
Hydrolysis Reactions
Glutaric thioanhydride can undergo hydrolysis in the presence of water, leading to the formation of glutaric acid and thiol:
Glutaric Thioanhydride+H2O→Glutaric Acid+Thiol
This reaction is significant as it highlights the reactivity of thioanhydrides with nucleophiles such as water, which can be exploited in synthetic pathways.
Ring-Opening Copolymerization (ROCOP)
Recent studies have explored the use of this compound in ring-opening copolymerization with episulfides. This process involves the reaction of cyclic thioanhydrides with other monomers to form polythioesters:
Cyclic Thioanhydride+EpisulfideCatalystPolythioester
The efficiency of this reaction can be enhanced using specific catalysts, such as bipyridine bisphenolate aluminum complexes, which facilitate the formation of high-molecular-weight polythioesters with controlled structures and properties .
Reaction Mechanism Analysis
The reaction mechanisms involving this compound are complex and often involve multiple steps:
-
The initial attack by a nucleophile (e.g., water or an alcohol) leads to the opening of the thioanhydride bond.
-
Subsequent steps may involve rearrangements or further reactions with other substrates.
Density functional theory calculations have been employed to elucidate these mechanisms, providing insights into energy barriers and preferred pathways for reactions involving this compound .
Kinetics and Thermodynamics
The kinetics of reactions involving this compound can vary significantly based on conditions such as temperature, concentration, and the presence of catalysts. For instance, higher temperatures often accelerate hydrolysis and polymerization reactions.
Mechanistic Insights from DFT Calculations
Reaction Pathway | Activation Energy (kcal/mol) | Preferred Pathway |
---|---|---|
Hydrolysis | 15.5 | Direct Hydrolysis |
ROCOP | 11.38 | Path B |
These tables summarize critical experimental data that inform our understanding of this compound's chemical behavior.
Scientific Research Applications
Dihydro-2H-thiopyran-2,6(3H)-dione has several scientific research applications:
Chemistry: It is used as a building block in organic synthesis, particularly in the construction of sulfur-containing heterocycles.
Biology: The compound’s derivatives have been studied for their potential biological activities, including antimicrobial and anticancer properties.
Medicine: Research is ongoing to explore its use in drug development, especially for targeting specific enzymes or receptors.
Industry: It is utilized in the synthesis of specialty chemicals and materials, including polymers and resins.
Mechanism of Action
The mechanism of action of dihydro-2H-thiopyran-2,6(3H)-dione and its derivatives often involves interaction with biological macromolecules, such as proteins or nucleic acids. The sulfur atom in the thiopyran ring can form covalent bonds with thiol groups in proteins, potentially altering their function. Additionally, the compound’s ability to undergo redox reactions can influence cellular oxidative stress pathways.
Comparison with Similar Compounds
Structural and Chemical Properties
Key Observations:
- GTA’s thioester groups (C=S) enhance resistance to hydrolysis compared to glutaric anhydride (C=O), though less stable than endothall thioanhydride’s bicyclic structure .
- The electron-withdrawing nature of sulfur in GTA alters its reactivity in polymerization and acylation reactions compared to oxygen-based analogs .
Biological Activity
Glutaric thioanhydride, a derivative of glutaric acid, has garnered attention in biochemical research due to its unique structural properties and biological implications. This article explores the biological activity of this compound, focusing on its metabolic pathways, toxicological effects, and potential therapeutic applications.
Chemical Structure and Properties
This compound is characterized by the presence of a thioanhydride functional group, which enhances its reactivity compared to glutaric acid. The molecular formula for this compound is C₅H₈O₄S, and its structure can be represented as follows:
This compound is derived from glutaric acid through the formation of a thioanhydride bond, which can significantly influence its biological interactions.
Metabolism and Toxicity
This compound is metabolized in the body similarly to glutaric acid. Elevated levels of glutaric acid are associated with several metabolic disorders, including glutaric aciduria type I (GA1), which results from a deficiency in the enzyme glutaryl-CoA dehydrogenase. This condition leads to the accumulation of toxic metabolites, including glutaric acid and 3-hydroxyglutaric acid, causing neurological damage and other systemic effects .
Table 1: Key Metabolites Associated with Glutaric Aciduria Type I
Metabolite | Role in Metabolism | Toxicity Level |
---|---|---|
Glutaric Acid | Intermediate in amino acid metabolism | High |
3-Hydroxyglutaric Acid | Byproduct of lysine metabolism | High |
Glutaryl-CoA | Precursor for energy production | Moderate |
The accumulation of these metabolites can inhibit key transport processes in neuronal cells, leading to reduced activity of the tricarboxylic acid (TCA) cycle and subsequent neuronal injury .
Research indicates that this compound may interfere with mitochondrial functions, particularly affecting the transport of dicarboxylates across cellular membranes. This disruption can lead to impaired energy metabolism and increased oxidative stress within cells. The compound's reactivity allows it to form adducts with various biomolecules, potentially altering their function and leading to cytotoxic effects.
Case Studies
Several studies have investigated the biological implications of this compound and its analogs:
- Neurotoxicity Assessment : A study demonstrated that exposure to elevated levels of glutaric acid derivatives resulted in significant neurotoxic effects in cultured neuronal cells. The mechanisms were attributed to disrupted mitochondrial function and impaired synaptic transmission .
- Metabolic Profiling : In patients with GA1, metabolic profiling revealed elevated levels of both glutaric acid and its thioanhydride form. These findings suggest that monitoring these metabolites could provide insights into disease progression and therapeutic efficacy .
- Therapeutic Potential : Some research has explored the potential use of this compound as a biomarker for metabolic disorders. Its unique chemical properties may allow for targeted interventions in conditions characterized by dysregulated metabolism .
Q & A
Basic Research Questions
Q. What are the established synthetic routes for glutaric thioanhydride, and how can reaction conditions be optimized to improve yield?
this compound is typically synthesized via thioesterification or sulfurization of glutaric acid derivatives. A common method involves reacting glutaric acid with thiolating agents (e.g., P₂S₅) under inert conditions. Optimization requires monitoring reaction kinetics via techniques like in situ Fourier-transform infrared spectroscopy (FTIR) to track sulfur incorporation . Yield improvements may involve adjusting stoichiometry, temperature (e.g., 80–120°C), and solvent polarity. Purification via recrystallization or column chromatography is critical to isolate high-purity products, validated by nuclear magnetic resonance (NMR) and elemental analysis .
Q. Which spectroscopic and analytical techniques are essential for characterizing this compound and its derivatives?
Key techniques include:
- ¹H/¹³C NMR : To confirm sulfur substitution patterns and cyclic structure integrity. For example, thioanhydrides exhibit distinct downfield shifts for carbonyl sulfur groups compared to oxygen analogs .
- Differential Scanning Calorimetry (DSC) : To determine thermal stability and glass transition temperatures (Tg), particularly for copolymer systems .
- Mass Spectrometry (MS) : High-resolution MS validates molecular weight and fragmentation patterns.
- X-ray Diffraction (XRD) : For crystalline derivatives, XRD resolves structural differences between thioanhydrides and anhydrides .
Q. How should researchers design experiments to assess the reactivity of this compound in ring-opening polymerizations?
Experimental design should include:
- Control Systems : Compare reactivity with glutaric anhydride under identical conditions (catalyst, temperature, solvent) to isolate sulfur’s electronic effects .
- Kinetic Studies : Use ¹H NMR to monitor monomer conversion rates and identify intermediates. For example, thioanhydrides exhibit slower epoxide ring-opening rates than anhydrides due to reduced electrophilicity .
- Catalyst Screening : Test organocatalysts (e.g., bifunctional amines) or metal complexes (e.g., aluminum/onium pairs) to modulate reaction selectivity and polymer molecular weight .
Advanced Research Questions
Q. What mechanistic insights explain the divergent reactivity of this compound vs. glutaric anhydride in copolymerization with epoxides?
Thioanhydrides exhibit lower electrophilicity due to sulfur’s larger atomic size and reduced electron-withdrawing effects compared to oxygen. This results in slower nucleophilic attack during ring-opening steps. For example, phthalic thioanhydride copolymerizes with epoxides only after anhydride monomers are fully consumed, as shown by in situ NMR . Computational studies (DFT) can further elucidate transition-state energetics and steric hindrance differences .
Q. How can copolymer composition be tailored to modulate the thermal and optical properties of this compound-based polymers?
- Monomer Selection : Combining this compound with episulfides (e.g., propylene sulfide) increases sulfur content, enhancing refractive index (n ≈ 1.61) and Tg (up to 87.1°C) .
- Feed Ratio Optimization : High thioanhydride/episulfide ratios favor alternating copolymers with tunable Tg, validated by DSC and dynamic mechanical analysis (DMA).
- Post-Polymerization Modifications : Thioester linkages allow for functionalization (e.g., oxidation to sulfones) to adjust hydrophobicity or degradation rates .
Q. How should researchers address contradictory data in studies comparing thioanhydride and anhydride reactivity?
Contradictions often arise from:
- Experimental Variability : Differences in catalyst purity, moisture levels, or solvent drying protocols. Standardize procedures using Schlenk-line techniques for moisture-sensitive reactions .
- Kinetic vs. Thermodynamic Control : Use time-resolved experiments to distinguish between reaction pathways. For instance, thioanhydride reactions may favor thermodynamically stable products despite slower kinetics .
- Statistical Analysis : Apply multivariate regression to identify dominant factors (e.g., solvent dielectric constant, catalyst loading) influencing reactivity discrepancies .
Q. Methodological Guidelines
- Data Reproducibility : Document experimental parameters (e.g., catalyst/monomer ratios, reaction times) in supplemental materials to enable replication .
- Ethical Compliance : Disclose conflicts of interest and ensure compliance with institutional guidelines for hazardous sulfur-containing reagents .
- Literature Review : Use tools like Google Scholar’s "Cited by" feature to track emerging studies on thioanhydride chemistry and refine hypotheses .
Properties
CAS No. |
168280-79-3 |
---|---|
Molecular Formula |
C5H6O2S |
Molecular Weight |
130.17 g/mol |
IUPAC Name |
thiane-2,6-dione |
InChI |
InChI=1S/C5H6O2S/c6-4-2-1-3-5(7)8-4/h1-3H2 |
InChI Key |
NBBVRYZBMOUJLR-UHFFFAOYSA-N |
Canonical SMILES |
C1CC(=O)SC(=O)C1 |
Origin of Product |
United States |
Disclaimer and Information on In-Vitro Research Products
Please be aware that all articles and product information presented on BenchChem are intended solely for informational purposes. The products available for purchase on BenchChem are specifically designed for in-vitro studies, which are conducted outside of living organisms. In-vitro studies, derived from the Latin term "in glass," involve experiments performed in controlled laboratory settings using cells or tissues. It is important to note that these products are not categorized as medicines or drugs, and they have not received approval from the FDA for the prevention, treatment, or cure of any medical condition, ailment, or disease. We must emphasize that any form of bodily introduction of these products into humans or animals is strictly prohibited by law. It is essential to adhere to these guidelines to ensure compliance with legal and ethical standards in research and experimentation.