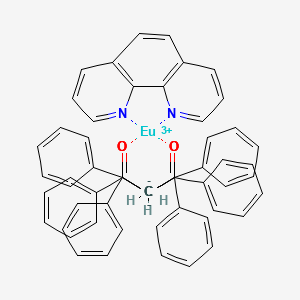
1,3-Diphenylpropane-1,3-dione;europium(3+);1,10-phenanthroline
- Click on QUICK INQUIRY to receive a quote from our team of experts.
- With the quality product at a COMPETITIVE price, you can focus more on your research.
Overview
Description
The compound “1,3-Diphenylpropane-1,3-dione;europium(3+);1,10-phenanthroline” is a coordination complex that consists of 1,3-diphenylpropane-1,3-dione, europium(3+), and 1,10-phenanthroline. This complex is known for its luminescent properties and is widely used in various scientific research applications, particularly in the fields of chemistry and materials science .
Preparation Methods
Synthetic Routes and Reaction Conditions
1,3-Diphenylpropane-1,3-dione can be synthesized through the Claisen condensation reaction between benzaldehyde and acetophenone in the presence of a base such as sodium ethoxide . The reaction is typically carried out under reflux conditions, and the product is purified through recrystallization.
Europium(3+) complexes with 1,3-diphenylpropane-1,3-dione and 1,10-phenanthroline can be prepared by mixing the ligands with europium chloride in a suitable solvent such as ethanol. The reaction mixture is stirred at room temperature for several hours, and the resulting complex is isolated by filtration and purified by recrystallization .
Industrial Production Methods
Industrial production of this complex involves large-scale synthesis of the individual components followed by their coordination. The process is optimized for high yield and purity, often involving automated systems for mixing, reaction control, and purification .
Chemical Reactions Analysis
Types of Reactions
1,3-Diphenylpropane-1,3-dione undergoes various chemical reactions, including oxidation, reduction, and substitution .
Common Reagents and Conditions
Reduction: Reducing agents like sodium borohydride can reduce the carbonyl groups to alcohols.
Substitution: Halogenation reactions can be carried out using halogenating agents like bromine or chlorine under controlled conditions.
Major Products
Oxidation: α-Iodo derivatives of 1,3-diphenylpropane-1,3-dione.
Reduction: Corresponding alcohols.
Substitution: Halogenated derivatives.
Scientific Research Applications
1,3-Diphenylpropane-1,3-dione;europium(3+);1,10-phenanthroline complex is extensively used in scientific research due to its unique luminescent properties . Some of the key applications include:
Chemistry: Used as a luminescent probe in various chemical reactions and processes.
Biology: Employed in bioimaging and as a fluorescent marker in biological assays.
Medicine: Investigated for potential use in photodynamic therapy and as a diagnostic tool.
Mechanism of Action
The luminescent properties of the complex arise from the energy transfer between the ligands and the europium ion. Upon excitation, the ligands absorb energy and transfer it to the europium ion, which then emits light. This process involves several molecular orbitals and pathways, including the π-π* transitions in the ligands and the f-f transitions in the europium ion .
Comparison with Similar Compounds
Similar Compounds
- 1-Phenyl-1,3-butanedione
- 2,2,6,6-Tetramethyl-3,5-heptanedione
- 4,4,4-Trifluoro-1-phenyl-1,3-butanedione
- Hexafluoroacetylacetone
Uniqueness
The 1,3-Diphenylpropane-1,3-dione;europium(3+);1,10-phenanthroline complex is unique due to its high luminescence efficiency and stability. The combination of 1,3-diphenylpropane-1,3-dione and 1,10-phenanthroline as ligands provides a synergistic effect, enhancing the overall luminescent properties of the europium ion .
Properties
Molecular Formula |
C57H41EuN2O6 |
---|---|
Molecular Weight |
1001.9 g/mol |
IUPAC Name |
1,3-diphenylpropane-1,3-dione;europium(3+);1,10-phenanthroline |
InChI |
InChI=1S/3C15H11O2.C12H8N2.Eu/c3*16-14(12-7-3-1-4-8-12)11-15(17)13-9-5-2-6-10-13;1-3-9-5-6-10-4-2-8-14-12(10)11(9)13-7-1;/h3*1-11H;1-8H;/q3*-1;;+3 |
InChI Key |
WXUAPKMSUYUWBD-UHFFFAOYSA-N |
Canonical SMILES |
C1=CC=C(C=C1)C(=O)[CH-]C(=O)C2=CC=CC=C2.C1=CC=C(C=C1)C(=O)[CH-]C(=O)C2=CC=CC=C2.C1=CC=C(C=C1)C(=O)[CH-]C(=O)C2=CC=CC=C2.C1=CC2=C(C3=C(C=CC=N3)C=C2)N=C1.[Eu+3] |
Origin of Product |
United States |
Disclaimer and Information on In-Vitro Research Products
Please be aware that all articles and product information presented on BenchChem are intended solely for informational purposes. The products available for purchase on BenchChem are specifically designed for in-vitro studies, which are conducted outside of living organisms. In-vitro studies, derived from the Latin term "in glass," involve experiments performed in controlled laboratory settings using cells or tissues. It is important to note that these products are not categorized as medicines or drugs, and they have not received approval from the FDA for the prevention, treatment, or cure of any medical condition, ailment, or disease. We must emphasize that any form of bodily introduction of these products into humans or animals is strictly prohibited by law. It is essential to adhere to these guidelines to ensure compliance with legal and ethical standards in research and experimentation.