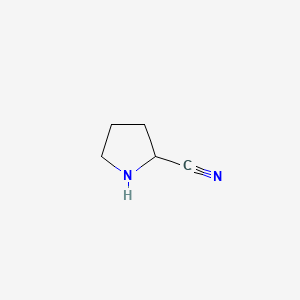
Pyrrolidine-2-carbonitrile
Overview
Description
Pyrrolidine-2-carbonitrile is a synthetic organic compound . It is a derivative of the five-membered pyrrolidine ring, which is one of the nitrogen heterocycles widely used by medicinal chemists to obtain compounds for the treatment of human diseases .
Synthesis Analysis
An alternative and practical synthesis of (S)-1-(2-chloroacetyl)pyrrolidine-2-carbonitrile was achieved. The reaction of L-proline with chloroacetyl chloride was followed by conversion of the carboxylic acid moiety of the resulting N-acylated product into the carbonitrile via the corresponding amide intermediate .Molecular Structure Analysis
The five-membered pyrrolidine ring is characterized by sp3-hybridization, contributing to the stereochemistry of the molecule, and increased three-dimensional (3D) coverage due to the non-planarity of the ring .Chemical Reactions Analysis
Pyrrolidine and its derivatives, including pyrrolizines, pyrrolidine-2-one, pyrrolidine-2,5-diones, and prolinol, are incorporated in bioactive molecules with target selectivity .Physical And Chemical Properties Analysis
Pyrrolidine-2-carbonitrile is a colorless, crystalline solid that is soluble in water and ethanol.Scientific Research Applications
Dipeptidyl Peptidase IV Inhibitors
Pyrrolidine-2-carbonitrile is a key intermediate in the synthesis of dipeptidyl peptidase IV (DPP-IV) inhibitors . DPP-IV inhibitors are emerging as a mechanism-based approach for the treatment of type-II diabetes . They work by extending the half-life of endogenously secreted glucagon-like peptide-1 (GLP-1), which enhances insulin secretion and improves glucose tolerance .
Organic Synthesis
(2S)-Pyrrolidine-2-carbonitrile serves as a valuable building block in organic synthesis. The presence of both a nitrogen atom and a nitrile group allows for various transformations, making it a versatile intermediate for the synthesis of complex molecules.
Synthesis of Vildagliptin
The synthesized pyrrolidine derivative from (S)-1-(2-chloroacetyl)pyrrolidine-2-carbonitrile is utilized to prepare DPP-IV inhibitor Vildagliptin . Vildagliptin is a treatment option for type-II diabetes .
Synthesis of Other DPP-IV Inhibitors
Because of its key role in DPP-IV inhibition, the 2 (S)-cyanopyrrolidine moiety, which can be derived from pyrrolidine-2-carbonitrile, has been found to be an integral part of many DPP-IV inhibitors .
Treatment of Type-II Diabetes
Inhibition of DPP-IV extends the half-life of endogenously secreted GLP-1, which in turn enhances insulin secretion and improves the glucose tolerance . DPP-IV inhibitors offer several potential advantages over existing therapies including decreased risk of hypoglycemia, potential for weight loss, and the potential for regeneration and differentiation of pancreatic β-cells .
Pharmaceutical Research
Pyrrolidine-2-carbonitrile is used in intense pharmaceutical research for the discovery of several DPP-IV inhibitors . Some of these inhibitors have entered clinical development recently .
Mechanism of Action
Target of Action
Pyrrolidine-2-carbonitrile is a complex molecule with a wide range of potential targets. . The pyrrolidine ring, a key component of Pyrrolidine-2-carbonitrile, is widely used by medicinal chemists to obtain compounds for the treatment of human diseases .
Mode of Action
The different stereoisomers and the spatial orientation of substituents can lead to a different biological profile of drug candidates, due to the different binding mode to enantioselective proteins .
Safety and Hazards
Pyrrolidine-2-carbonitrile should be handled with care. Avoid dust formation, breathing mist, gas or vapors, and contact with skin and eyes. Use personal protective equipment, ensure adequate ventilation, and remove all sources of ignition . It may cause skin irritation, serious eye irritation, and respiratory irritation. It is harmful if swallowed, in contact with skin, or if inhaled .
Future Directions
properties
IUPAC Name |
pyrrolidine-2-carbonitrile | |
---|---|---|
Source | PubChem | |
URL | https://pubchem.ncbi.nlm.nih.gov | |
Description | Data deposited in or computed by PubChem | |
InChI |
InChI=1S/C5H8N2/c6-4-5-2-1-3-7-5/h5,7H,1-3H2 | |
Source | PubChem | |
URL | https://pubchem.ncbi.nlm.nih.gov | |
Description | Data deposited in or computed by PubChem | |
InChI Key |
ALSCEGDXFJIYES-UHFFFAOYSA-N | |
Source | PubChem | |
URL | https://pubchem.ncbi.nlm.nih.gov | |
Description | Data deposited in or computed by PubChem | |
Canonical SMILES |
C1CC(NC1)C#N | |
Source | PubChem | |
URL | https://pubchem.ncbi.nlm.nih.gov | |
Description | Data deposited in or computed by PubChem | |
Molecular Formula |
C5H8N2 | |
Source | PubChem | |
URL | https://pubchem.ncbi.nlm.nih.gov | |
Description | Data deposited in or computed by PubChem | |
DSSTOX Substance ID |
DTXSID10405749 | |
Record name | Pyrrolidine-2-carbonitrile | |
Source | EPA DSSTox | |
URL | https://comptox.epa.gov/dashboard/DTXSID10405749 | |
Description | DSSTox provides a high quality public chemistry resource for supporting improved predictive toxicology. | |
Molecular Weight |
96.13 g/mol | |
Source | PubChem | |
URL | https://pubchem.ncbi.nlm.nih.gov | |
Description | Data deposited in or computed by PubChem | |
CAS RN |
5626-49-3 | |
Record name | Pyrrolidine-2-carbonitrile | |
Source | EPA DSSTox | |
URL | https://comptox.epa.gov/dashboard/DTXSID10405749 | |
Description | DSSTox provides a high quality public chemistry resource for supporting improved predictive toxicology. | |
Retrosynthesis Analysis
AI-Powered Synthesis Planning: Our tool employs the Template_relevance Pistachio, Template_relevance Bkms_metabolic, Template_relevance Pistachio_ringbreaker, Template_relevance Reaxys, Template_relevance Reaxys_biocatalysis model, leveraging a vast database of chemical reactions to predict feasible synthetic routes.
One-Step Synthesis Focus: Specifically designed for one-step synthesis, it provides concise and direct routes for your target compounds, streamlining the synthesis process.
Accurate Predictions: Utilizing the extensive PISTACHIO, BKMS_METABOLIC, PISTACHIO_RINGBREAKER, REAXYS, REAXYS_BIOCATALYSIS database, our tool offers high-accuracy predictions, reflecting the latest in chemical research and data.
Strategy Settings
Precursor scoring | Relevance Heuristic |
---|---|
Min. plausibility | 0.01 |
Model | Template_relevance |
Template Set | Pistachio/Bkms_metabolic/Pistachio_ringbreaker/Reaxys/Reaxys_biocatalysis |
Top-N result to add to graph | 6 |
Feasible Synthetic Routes
Q & A
Q1: What is the molecular formula and weight of pyrrolidine-2-carbonitrile?
A1: The molecular formula of pyrrolidine-2-carbonitrile is C5H8N2, and its molecular weight is 96.13 g/mol.
Q2: What spectroscopic data is available for characterizing pyrrolidine-2-carbonitrile?
A3: Characterization data often includes 1H NMR, 13C NMR, IR, and mass spectrometry. For example, one study utilized 1H NMR and MS to confirm the structure of synthesized (S)-1-(2-chloroacetyl)pyrrolidine-2-carbonitrile, a key intermediate in the synthesis of DPP-4 inhibitors. []
Q3: Are there any specific stability concerns associated with pyrrolidine-2-carbonitrile derivatives?
A4: Stability can vary depending on the specific substituents on the pyrrolidine-2-carbonitrile core. Research has focused on improving the stability of these derivatives, particularly under various storage conditions. [] For instance, one study explored different formulation strategies to enhance the stability of Vildagliptin, a DPP-4 inhibitor containing a pyrrolidine-2-carbonitrile moiety. []
Q4: Does pyrrolidine-2-carbonitrile exhibit any catalytic properties?
A4: While pyrrolidine-2-carbonitrile itself might not be recognized for its catalytic activity, its derivatives have been explored as organocatalysts in specific organic reactions.
Q5: How is computational chemistry used in the development of pyrrolidine-2-carbonitrile-based drugs?
A6: Computational tools like docking studies and 3D-QSAR are frequently employed to predict the binding affinity and activity of novel pyrrolidine-2-carbonitrile derivatives as potential DPP-4 inhibitors. [] These techniques help prioritize the synthesis and biological evaluation of promising candidates.
Q6: How do structural modifications to the pyrrolidine-2-carbonitrile core affect DPP-4 inhibitory activity?
A7: Extensive SAR studies have revealed the impact of substituents on the pyrrolidine ring and the nature of the group attached to the nitrile on the potency, selectivity, and pharmacokinetic properties of DPP-4 inhibitors. [, ] Researchers have explored various heterocyclic rings, aromatic groups, and substituted alkyl chains to optimize these parameters.
Q7: What are some common formulation strategies employed to improve the stability and bioavailability of pyrrolidine-2-carbonitrile-based drugs?
A8: Formulation approaches, including the use of suitable excipients, particle size reduction, and different dosage forms like tablets or capsules, are crucial for optimizing the stability, solubility, and consequently, the bioavailability of pyrrolidine-2-carbonitrile-containing drugs. []
Q8: What is the typical pharmacokinetic profile of Vildagliptin?
A9: Vildagliptin exhibits good oral bioavailability and a relatively long half-life, allowing for once-daily dosing. [] It is primarily metabolized by hydrolysis, and its metabolites are mainly excreted in the feces. []
Q9: What animal models are commonly used to assess the efficacy of DPP-4 inhibitors?
A10: Spontaneously Hypertensive Rats-Streptozotocin (SHR-STZ) animal models are frequently employed to evaluate the antidiabetic potential of novel pyrrolidine-2-carbonitrile derivatives. []
Q10: What analytical techniques are used to characterize and quantify pyrrolidine-2-carbonitrile and its derivatives?
A12: Various analytical methods, including HPLC, have been developed for the estimation of Vildagliptin in bulk and tablet dosage forms. [] These methods are validated according to ICH guidelines to ensure accuracy, precision, and specificity. []
Disclaimer and Information on In-Vitro Research Products
Please be aware that all articles and product information presented on BenchChem are intended solely for informational purposes. The products available for purchase on BenchChem are specifically designed for in-vitro studies, which are conducted outside of living organisms. In-vitro studies, derived from the Latin term "in glass," involve experiments performed in controlled laboratory settings using cells or tissues. It is important to note that these products are not categorized as medicines or drugs, and they have not received approval from the FDA for the prevention, treatment, or cure of any medical condition, ailment, or disease. We must emphasize that any form of bodily introduction of these products into humans or animals is strictly prohibited by law. It is essential to adhere to these guidelines to ensure compliance with legal and ethical standards in research and experimentation.