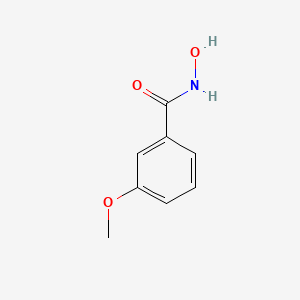
m-Methoxybenzohydroxamic acid
- Click on QUICK INQUIRY to receive a quote from our team of experts.
- With the quality product at a COMPETITIVE price, you can focus more on your research.
Overview
Description
m-Methoxybenzohydroxamic acid is an organic compound belonging to the class of hydroxamic acids These compounds are characterized by the presence of a hydroxylamine group (-NH-OH) attached to a carbonyl group (C=O) this compound is specifically distinguished by a methoxy group (-OCH3) attached to the benzene ring in the meta position relative to the hydroxamic acid group
Preparation Methods
Synthetic Routes and Reaction Conditions
The synthesis of m-Methoxybenzohydroxamic acid typically involves the reaction of m-methoxybenzoyl chloride with hydroxylamine hydrochloride in the presence of a base such as sodium bicarbonate. The reaction is carried out in an ice-cooled ethanol solution to control the temperature and prevent side reactions. The resulting product is then purified through recrystallization .
Industrial Production Methods
Industrial production methods for this compound are similar to laboratory synthesis but are scaled up to accommodate larger quantities. The process involves the same reactants and conditions but utilizes larger reaction vessels and more efficient purification techniques to ensure high yield and purity .
Chemical Reactions Analysis
Types of Reactions
m-Methoxybenzohydroxamic acid undergoes various chemical reactions, including:
Oxidation: The compound can be oxidized to form corresponding nitroso derivatives.
Reduction: Reduction reactions can convert the hydroxamic acid group to an amide group.
Substitution: The methoxy group can be substituted with other functional groups under appropriate conditions.
Common Reagents and Conditions
Common reagents used in these reactions include oxidizing agents like potassium permanganate for oxidation, reducing agents like lithium aluminum hydride for reduction, and nucleophiles for substitution reactions. The reactions are typically carried out under controlled temperatures and in suitable solvents to ensure selectivity and yield .
Major Products Formed
The major products formed from these reactions include nitroso derivatives, amides, and substituted benzohydroxamic acids.
Scientific Research Applications
m-Methoxybenzohydroxamic acid has several scientific research applications:
Mechanism of Action
The mechanism of action of m-Methoxybenzohydroxamic acid involves its ability to chelate metal ions and inhibit enzyme activity. The hydroxamic acid group binds to metal ions, forming stable complexes that can interfere with the function of metalloenzymes. This chelation disrupts the enzyme’s active site, inhibiting its activity. The compound’s methoxy group also contributes to its binding affinity and specificity .
Comparison with Similar Compounds
Similar Compounds
- N-methyl-o-methoxybenzohydroxamic acid
- N-methyl-p-methoxybenzohydroxamic acid
- 2-Methoxybenzohydroxamic acid
Comparison
m-Methoxybenzohydroxamic acid is unique due to the position of the methoxy group on the benzene ring. This positional difference affects its chemical reactivity, binding affinity, and biological activity compared to its ortho and para counterparts. For example, the meta position may result in different steric and electronic effects, influencing the compound’s ability to form complexes and inhibit enzymes .
Properties
Molecular Formula |
C8H9NO3 |
---|---|
Molecular Weight |
167.16 g/mol |
IUPAC Name |
N-hydroxy-3-methoxybenzamide |
InChI |
InChI=1S/C8H9NO3/c1-12-7-4-2-3-6(5-7)8(10)9-11/h2-5,11H,1H3,(H,9,10) |
InChI Key |
DVUJVMMFEBNGPA-UHFFFAOYSA-N |
Canonical SMILES |
COC1=CC=CC(=C1)C(=O)NO |
Origin of Product |
United States |
Disclaimer and Information on In-Vitro Research Products
Please be aware that all articles and product information presented on BenchChem are intended solely for informational purposes. The products available for purchase on BenchChem are specifically designed for in-vitro studies, which are conducted outside of living organisms. In-vitro studies, derived from the Latin term "in glass," involve experiments performed in controlled laboratory settings using cells or tissues. It is important to note that these products are not categorized as medicines or drugs, and they have not received approval from the FDA for the prevention, treatment, or cure of any medical condition, ailment, or disease. We must emphasize that any form of bodily introduction of these products into humans or animals is strictly prohibited by law. It is essential to adhere to these guidelines to ensure compliance with legal and ethical standards in research and experimentation.