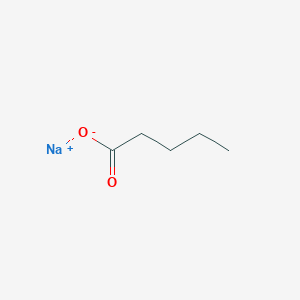
Sodium pentanoate
- Click on QUICK INQUIRY to receive a quote from our team of experts.
- With the quality product at a COMPETITIVE price, you can focus more on your research.
Overview
Description
It is a white crystalline or granular substance that is soluble in water . Sodium pentanoate is primarily used in chemical laboratories as a component of neutral laboratory buffers to control the pH of solutions. Additionally, it finds applications in the pharmaceutical and food industries as a preservative and flavoring agent .
Preparation Methods
Sodium pentanoate is typically synthesized by reacting valeric acid with sodium hydroxide. The process involves dissolving valeric acid in an appropriate amount of water and then slowly adding sodium hydroxide solution. Once the reaction is complete, the pure sodium valerate crystals are filtered out . Industrial production methods follow a similar approach, ensuring safety measures to avoid contact with skin, eyes, and inhalation of dust .
Chemical Reactions Analysis
Sodium pentanoate undergoes various chemical reactions typical of carboxylate salts. Some of the key reactions include:
Neutralization Reactions: This compound can react with acids to form valeric acid and the corresponding sodium salt of the acid used.
Esterification: It can react with alcohols in the presence of an acid catalyst to form esters.
Amidation: This compound can react with amines to form amides.
Common reagents used in these reactions include acids (e.g., hydrochloric acid), alcohols (e.g., ethanol), and amines (e.g., ammonia). The major products formed from these reactions are valeric acid, esters, and amides .
Scientific Research Applications
Sodium pentanoate has a wide range of applications in scientific research:
Mechanism of Action
The mechanism of action of sodium valerate involves its role as a short-chain fatty acid. It has a similar structure to γ-aminobutyric acid (GABA) and can increase GABA levels in the body. This increase in GABA levels is associated with various physiological effects, including reduced alcohol intake and improved anxiety-like behavior in animal models . Sodium pentanoate also influences gene expression related to potassium voltage-gated channels, inflammation, and psychological behaviors .
Comparison with Similar Compounds
Sodium pentanoate is part of the short-chain fatty acids (SCFAs) family, which includes compounds like acetate, propionate, butyrate, isovalerate, and isobutyrate . Compared to these similar compounds, sodium valerate has unique properties such as its ability to promote intestinal barrier function and its potential therapeutic effects on alcohol intake and anxiety . Other SCFAs like butyrate are well-known for their gut health benefits, but sodium valerate offers a broader range of effective concentrations and additional physiological effects .
Properties
CAS No. |
6106-41-8 |
---|---|
Molecular Formula |
C5H10NaO2 |
Molecular Weight |
125.12 g/mol |
IUPAC Name |
sodium;pentanoate |
InChI |
InChI=1S/C5H10O2.Na/c1-2-3-4-5(6)7;/h2-4H2,1H3,(H,6,7); |
InChI Key |
RWSZDLPYUAAMDO-UHFFFAOYSA-N |
Isomeric SMILES |
CCCCC(=O)[O-].[Na+] |
SMILES |
CCCCC(=O)[O-].[Na+] |
Canonical SMILES |
CCCCC(=O)O.[Na] |
6106-41-8 | |
Related CAS |
109-52-4 (Parent) |
Synonyms |
Pentanoic Acid Sodium Salt; Sodium n-Valerate; Sodium Pentanoate; Sodium Valerate |
Origin of Product |
United States |
Retrosynthesis Analysis
AI-Powered Synthesis Planning: Our tool employs the Template_relevance Pistachio, Template_relevance Bkms_metabolic, Template_relevance Pistachio_ringbreaker, Template_relevance Reaxys, Template_relevance Reaxys_biocatalysis model, leveraging a vast database of chemical reactions to predict feasible synthetic routes.
One-Step Synthesis Focus: Specifically designed for one-step synthesis, it provides concise and direct routes for your target compounds, streamlining the synthesis process.
Accurate Predictions: Utilizing the extensive PISTACHIO, BKMS_METABOLIC, PISTACHIO_RINGBREAKER, REAXYS, REAXYS_BIOCATALYSIS database, our tool offers high-accuracy predictions, reflecting the latest in chemical research and data.
Strategy Settings
Precursor scoring | Relevance Heuristic |
---|---|
Min. plausibility | 0.01 |
Model | Template_relevance |
Template Set | Pistachio/Bkms_metabolic/Pistachio_ringbreaker/Reaxys/Reaxys_biocatalysis |
Top-N result to add to graph | 6 |
Feasible Synthetic Routes
Disclaimer and Information on In-Vitro Research Products
Please be aware that all articles and product information presented on BenchChem are intended solely for informational purposes. The products available for purchase on BenchChem are specifically designed for in-vitro studies, which are conducted outside of living organisms. In-vitro studies, derived from the Latin term "in glass," involve experiments performed in controlled laboratory settings using cells or tissues. It is important to note that these products are not categorized as medicines or drugs, and they have not received approval from the FDA for the prevention, treatment, or cure of any medical condition, ailment, or disease. We must emphasize that any form of bodily introduction of these products into humans or animals is strictly prohibited by law. It is essential to adhere to these guidelines to ensure compliance with legal and ethical standards in research and experimentation.