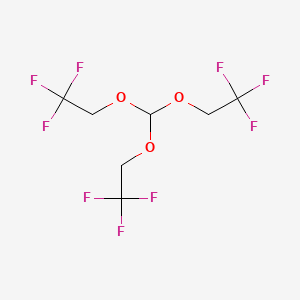
Tris(2,2,2-trifluoroethyl)orthoformate
Overview
Description
Tris(2,2,2-trifluoroethyl)orthoformate:
Mechanism of Action
Target of Action
Tris(2,2,2-trifluoroethyl)orthoformate is primarily targeted towards the lithium metal anode surface in lithium metal batteries . The lithium metal anode is a crucial component of these batteries, playing a significant role in their performance and efficiency.
Mode of Action
The interaction of this compound with its target involves exhibiting high concentration electrolyte behavior . This behavior is essential in maintaining the stability and functionality of the lithium metal batteries.
Biochemical Pathways
The biochemical pathways affected by this compound are primarily related to the electrochemical processes in lithium metal batteries . The compound’s action can influence the formation of stable Solid Electrolyte Interface (SEI) layers, which act as kinetic barriers between the electrolyte and the electrodes . These layers limit the continuous side reactions on both the lithium metal anode and high-voltage cathode during repeated cycling .
Pharmacokinetics
The compound’s presence and concentration can significantly affect the performance and efficiency of these batteries .
Result of Action
The molecular and cellular effects of this compound’s action are manifested in the improved performance and stability of lithium metal batteries . By influencing the formation of stable SEI layers, the compound helps limit unwanted side reactions, thereby enhancing the battery’s overall efficiency .
Action Environment
Environmental factors can influence the action, efficacy, and stability of this compound. For instance, contamination with oxidizing agents such as nitrates, oxidizing acids, chlorine bleaches, pool chlorine, etc., should be avoided as ignition may result .
Preparation Methods
Synthetic Routes and Reaction Conditions: Industrial Production Methods:
Chemical Reactions Analysis
Types of Reactions: Tris(2,2,2-trifluoroethyl)orthoformate undergoes various chemical reactions, including:
Substitution Reactions: It can react with nucleophiles to form substituted products[][1].
Hydrolysis: In the presence of water, it can hydrolyze to form trifluoroethanol and orthoformic acid[][1].
Common Reagents and Conditions:
Nucleophiles: Common nucleophiles used in substitution reactions include amines and alcohols[][1].
Catalysts: Acidic or basic catalysts are often used to facilitate these reactions[][1].
Major Products:
Substituted Products: Depending on the nucleophile used, various substituted derivatives of this compound can be formed[][1].
Hydrolysis Products: Trifluoroethanol and orthoformic acid are the primary products of hydrolysis[][1].
Scientific Research Applications
Chemistry: Biology and Medicine: Industry:
Comparison with Similar Compounds
- Tris(2,2,2-trifluoroethoxy)methane
- Tris(2,2,2-trifluoroethyl)orthoacetate
- Tris(2,2,2-trifluoroethyl)orthopropionate [1][1]
Properties
IUPAC Name |
2-[bis(2,2,2-trifluoroethoxy)methoxy]-1,1,1-trifluoroethane | |
---|---|---|
Source | PubChem | |
URL | https://pubchem.ncbi.nlm.nih.gov | |
Description | Data deposited in or computed by PubChem | |
InChI |
InChI=1S/C7H7F9O3/c8-5(9,10)1-17-4(18-2-6(11,12)13)19-3-7(14,15)16/h4H,1-3H2 | |
Source | PubChem | |
URL | https://pubchem.ncbi.nlm.nih.gov | |
Description | Data deposited in or computed by PubChem | |
InChI Key |
IESBVSNCDNHMSL-UHFFFAOYSA-N | |
Source | PubChem | |
URL | https://pubchem.ncbi.nlm.nih.gov | |
Description | Data deposited in or computed by PubChem | |
Canonical SMILES |
C(C(F)(F)F)OC(OCC(F)(F)F)OCC(F)(F)F | |
Source | PubChem | |
URL | https://pubchem.ncbi.nlm.nih.gov | |
Description | Data deposited in or computed by PubChem | |
Molecular Formula |
C7H7F9O3 | |
Source | PubChem | |
URL | https://pubchem.ncbi.nlm.nih.gov | |
Description | Data deposited in or computed by PubChem | |
DSSTOX Substance ID |
DTXSID30395037 | |
Record name | tris(Trifluoroethoxy)methane | |
Source | EPA DSSTox | |
URL | https://comptox.epa.gov/dashboard/DTXSID30395037 | |
Description | DSSTox provides a high quality public chemistry resource for supporting improved predictive toxicology. | |
Molecular Weight |
310.11 g/mol | |
Source | PubChem | |
URL | https://pubchem.ncbi.nlm.nih.gov | |
Description | Data deposited in or computed by PubChem | |
CAS No. |
58244-27-2 | |
Record name | Ethane, 1,1′,1′′-[methylidynetris(oxy)]tris[2,2,2-trifluoro- | |
Source | CAS Common Chemistry | |
URL | https://commonchemistry.cas.org/detail?cas_rn=58244-27-2 | |
Description | CAS Common Chemistry is an open community resource for accessing chemical information. Nearly 500,000 chemical substances from CAS REGISTRY cover areas of community interest, including common and frequently regulated chemicals, and those relevant to high school and undergraduate chemistry classes. This chemical information, curated by our expert scientists, is provided in alignment with our mission as a division of the American Chemical Society. | |
Explanation | The data from CAS Common Chemistry is provided under a CC-BY-NC 4.0 license, unless otherwise stated. | |
Record name | tris(Trifluoroethoxy)methane | |
Source | EPA DSSTox | |
URL | https://comptox.epa.gov/dashboard/DTXSID30395037 | |
Description | DSSTox provides a high quality public chemistry resource for supporting improved predictive toxicology. | |
Record name | Tris(2,2,2-trifluoroethyl)orthoformate | |
Source | European Chemicals Agency (ECHA) | |
URL | https://echa.europa.eu/information-on-chemicals | |
Description | The European Chemicals Agency (ECHA) is an agency of the European Union which is the driving force among regulatory authorities in implementing the EU's groundbreaking chemicals legislation for the benefit of human health and the environment as well as for innovation and competitiveness. | |
Explanation | Use of the information, documents and data from the ECHA website is subject to the terms and conditions of this Legal Notice, and subject to other binding limitations provided for under applicable law, the information, documents and data made available on the ECHA website may be reproduced, distributed and/or used, totally or in part, for non-commercial purposes provided that ECHA is acknowledged as the source: "Source: European Chemicals Agency, http://echa.europa.eu/". Such acknowledgement must be included in each copy of the material. ECHA permits and encourages organisations and individuals to create links to the ECHA website under the following cumulative conditions: Links can only be made to webpages that provide a link to the Legal Notice page. | |
Retrosynthesis Analysis
AI-Powered Synthesis Planning: Our tool employs the Template_relevance Pistachio, Template_relevance Bkms_metabolic, Template_relevance Pistachio_ringbreaker, Template_relevance Reaxys, Template_relevance Reaxys_biocatalysis model, leveraging a vast database of chemical reactions to predict feasible synthetic routes.
One-Step Synthesis Focus: Specifically designed for one-step synthesis, it provides concise and direct routes for your target compounds, streamlining the synthesis process.
Accurate Predictions: Utilizing the extensive PISTACHIO, BKMS_METABOLIC, PISTACHIO_RINGBREAKER, REAXYS, REAXYS_BIOCATALYSIS database, our tool offers high-accuracy predictions, reflecting the latest in chemical research and data.
Strategy Settings
Precursor scoring | Relevance Heuristic |
---|---|
Min. plausibility | 0.01 |
Model | Template_relevance |
Template Set | Pistachio/Bkms_metabolic/Pistachio_ringbreaker/Reaxys/Reaxys_biocatalysis |
Top-N result to add to graph | 6 |
Feasible Synthetic Routes
Q1: How does TFEO contribute to the formation of a stable solid-electrolyte interphase (SEI) in Li-metal batteries?
A1: Research suggests that TFEO plays a crucial role in constructing a stable SEI, a critical factor for Li-metal battery performance. During the initial charging process, TFEO readily decomposes in the electron-rich environment near the Li-metal anode. [, ] This decomposition releases fluoride ions (F-) that contribute to the formation of a LiF-rich inorganic inner layer within the SEI. [] This LiF-rich layer is considered beneficial as it provides a robust barrier against further electrolyte decomposition and uncontrolled lithium dendrite growth. [] Additionally, the decomposition of TFEO produces unsaturated carbon compounds, like thiophene, which may enhance the SEI's conductivity, further improving battery performance. []
Q2: What are the key interactions between TFEO and other components in LHCEs?
A2: Computational studies have revealed that the interaction of TFEO with the solvent and salt influences the solvation structure within the LHCE. [] Notably, the solubility of LiFSI salt in the DME/TFEO mixed solvent is not solely determined by the dielectric constant but is significantly impacted by the molecular structure and the resulting solvation shell structure. [] Molecular dynamics simulations showed that despite the presence of TFEO, the Li+ solvation shells primarily consist of anions and solvent molecules, with minimal TFEO involvement. [] This suggests that TFEO contributes to forming localized high-concentration regions of the Li-salt and solvent while maintaining a less-coordinating environment around the Li+ ions, potentially beneficial for Li+ transport.
Q3: What are the decomposition pathways of TFEO in LHCEs under electron-rich conditions?
A3: DFT-ReaxFF hybrid molecular dynamics simulations have provided detailed insights into the decomposition mechanisms of TFEO in the presence of excess electrons. [] TFEO decomposition typically involves a six-electron transfer process, leading to the formation of F-, formate ions (HCOO-), and various fluorinated carbon species like CH2CF- and -OCH2CF3. [] This decomposition process is energetically favorable with relatively low activation barriers, explaining the observed rapid breakdown of TFEO during SEI formation. []
Q4: What analytical techniques are employed to study the SEI formation and TFEO decomposition in LHCEs?
A4: Researchers utilize a combination of computational and experimental techniques to study SEI formation and TFEO decomposition in LHCEs. Density functional theory (DFT) and classical molecular dynamics (MD) simulations help predict interactions, decomposition pathways, and SEI structure evolution. [, ] Experimentally, X-ray photoelectron spectroscopy (XPS) analysis is used to investigate the atomic composition and chemical states of the SEI layer, providing insights into the decomposition products of TFEO and other electrolyte components. [] Combining these approaches allows for a comprehensive understanding of the role of TFEO in LHCEs and its impact on battery performance.
Disclaimer and Information on In-Vitro Research Products
Please be aware that all articles and product information presented on BenchChem are intended solely for informational purposes. The products available for purchase on BenchChem are specifically designed for in-vitro studies, which are conducted outside of living organisms. In-vitro studies, derived from the Latin term "in glass," involve experiments performed in controlled laboratory settings using cells or tissues. It is important to note that these products are not categorized as medicines or drugs, and they have not received approval from the FDA for the prevention, treatment, or cure of any medical condition, ailment, or disease. We must emphasize that any form of bodily introduction of these products into humans or animals is strictly prohibited by law. It is essential to adhere to these guidelines to ensure compliance with legal and ethical standards in research and experimentation.