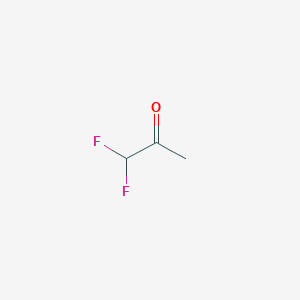
1,1-Difluoroacetone
Overview
Description
1,1-Difluoroacetone is an organic compound with the molecular formula C₃H₄F₂O. It is a colorless liquid with a distinct odor and is known for its reactivity due to the presence of two fluorine atoms attached to the carbonyl group. This compound is used primarily in research and development settings and has various applications in organic synthesis.
Mechanism of Action
Target of Action
1,1-Difluoroacetone is a chemical compound with the molecular formula C3H4F2O The primary targets of this compound are not well-documented in the literature
Biochemical Pathways
It’s known that the compound is used in the preparation of substituted thf amides as modulators of sodium channels This suggests that it may play a role in the modulation of sodium channels, which are crucial for the transmission of nerve impulses
Action Environment
Environmental factors can significantly influence the action, efficacy, and stability of a compound. For this compound, a cost-effective availability could revolutionize the current methodologies for fluoropyrazole ring synthesis, primarily due to its enhanced safety and environmental sustainability . .
Preparation Methods
Synthetic Routes and Reaction Conditions
1,1-Difluoroacetone can be synthesized through several methods. One common method involves the fluorination of acetone using a fluorinating agent such as sulfur tetrafluoride (SF₄) or diethylaminosulfur trifluoride (DAST). The reaction typically occurs under controlled conditions to ensure the selective introduction of fluorine atoms.
Industrial Production Methods
In an industrial setting, the production of this compound may involve the use of more scalable fluorination techniques, such as the use of hydrogen fluoride (HF) in the presence of a catalyst. The reaction conditions, including temperature and pressure, are optimized to maximize yield and purity.
Chemical Reactions Analysis
Types of Reactions
1,1-Difluoroacetone undergoes various chemical reactions, including:
Oxidation: It can be oxidized to form difluoroacetic acid.
Reduction: Reduction reactions can yield difluoroalcohols.
Substitution: The fluorine atoms can be substituted with other nucleophiles, leading to a variety of derivatives.
Common Reagents and Conditions
Oxidation: Common oxidizing agents include potassium permanganate (KMnO₄) and chromium trioxide (CrO₃).
Reduction: Reducing agents such as lithium aluminum hydride (LiAlH₄) or sodium borohydride (NaBH₄) are used.
Substitution: Nucleophiles like amines, thiols, and halides can react with this compound under appropriate conditions.
Major Products
Oxidation: Difluoroacetic acid.
Reduction: Difluoroalcohols.
Substitution: Various substituted derivatives depending on the nucleophile used.
Scientific Research Applications
1,1-Difluoroacetone has several applications in scientific research:
Chemistry: It is used as a building block in the synthesis of more complex fluorinated compounds.
Biology: It serves as a probe in studying enzyme mechanisms and metabolic pathways involving fluorinated substrates.
Medicine: Research into potential pharmaceutical applications, particularly in the development of enzyme inhibitors.
Industry: Used in the production of specialty chemicals and materials with unique properties due to the presence of fluorine atoms.
Comparison with Similar Compounds
Similar Compounds
1,3-Difluoroacetone: Another fluorinated acetone derivative with fluorine atoms at different positions.
1,1,1-Trifluoroacetone: Contains three fluorine atoms, leading to different reactivity and properties.
Fluoroacetone: A single fluorine atom, resulting in distinct chemical behavior.
Uniqueness
1,1-Difluoroacetone is unique due to the specific positioning of the fluorine atoms, which significantly influences its reactivity and the types of reactions it can undergo. This makes it a valuable compound in synthetic chemistry and research applications.
Properties
IUPAC Name |
1,1-difluoropropan-2-one | |
---|---|---|
Source | PubChem | |
URL | https://pubchem.ncbi.nlm.nih.gov | |
Description | Data deposited in or computed by PubChem | |
InChI |
InChI=1S/C3H4F2O/c1-2(6)3(4)5/h3H,1H3 | |
Source | PubChem | |
URL | https://pubchem.ncbi.nlm.nih.gov | |
Description | Data deposited in or computed by PubChem | |
InChI Key |
XHILZHAQBOLGFD-UHFFFAOYSA-N | |
Source | PubChem | |
URL | https://pubchem.ncbi.nlm.nih.gov | |
Description | Data deposited in or computed by PubChem | |
Canonical SMILES |
CC(=O)C(F)F | |
Source | PubChem | |
URL | https://pubchem.ncbi.nlm.nih.gov | |
Description | Data deposited in or computed by PubChem | |
Molecular Formula |
C3H4F2O | |
Source | PubChem | |
URL | https://pubchem.ncbi.nlm.nih.gov | |
Description | Data deposited in or computed by PubChem | |
DSSTOX Substance ID |
DTXSID30393018 | |
Record name | 1,1-difluoroacetone | |
Source | EPA DSSTox | |
URL | https://comptox.epa.gov/dashboard/DTXSID30393018 | |
Description | DSSTox provides a high quality public chemistry resource for supporting improved predictive toxicology. | |
Molecular Weight |
94.06 g/mol | |
Source | PubChem | |
URL | https://pubchem.ncbi.nlm.nih.gov | |
Description | Data deposited in or computed by PubChem | |
CAS No. |
431-05-0 | |
Record name | 1,1-Difluoro-2-propanone | |
Source | CAS Common Chemistry | |
URL | https://commonchemistry.cas.org/detail?cas_rn=431-05-0 | |
Description | CAS Common Chemistry is an open community resource for accessing chemical information. Nearly 500,000 chemical substances from CAS REGISTRY cover areas of community interest, including common and frequently regulated chemicals, and those relevant to high school and undergraduate chemistry classes. This chemical information, curated by our expert scientists, is provided in alignment with our mission as a division of the American Chemical Society. | |
Explanation | The data from CAS Common Chemistry is provided under a CC-BY-NC 4.0 license, unless otherwise stated. | |
Record name | 1,1-difluoroacetone | |
Source | EPA DSSTox | |
URL | https://comptox.epa.gov/dashboard/DTXSID30393018 | |
Description | DSSTox provides a high quality public chemistry resource for supporting improved predictive toxicology. | |
Record name | 1,1-Difluoroacetone | |
Source | European Chemicals Agency (ECHA) | |
URL | https://echa.europa.eu/information-on-chemicals | |
Description | The European Chemicals Agency (ECHA) is an agency of the European Union which is the driving force among regulatory authorities in implementing the EU's groundbreaking chemicals legislation for the benefit of human health and the environment as well as for innovation and competitiveness. | |
Explanation | Use of the information, documents and data from the ECHA website is subject to the terms and conditions of this Legal Notice, and subject to other binding limitations provided for under applicable law, the information, documents and data made available on the ECHA website may be reproduced, distributed and/or used, totally or in part, for non-commercial purposes provided that ECHA is acknowledged as the source: "Source: European Chemicals Agency, http://echa.europa.eu/". Such acknowledgement must be included in each copy of the material. ECHA permits and encourages organisations and individuals to create links to the ECHA website under the following cumulative conditions: Links can only be made to webpages that provide a link to the Legal Notice page. | |
Retrosynthesis Analysis
AI-Powered Synthesis Planning: Our tool employs the Template_relevance Pistachio, Template_relevance Bkms_metabolic, Template_relevance Pistachio_ringbreaker, Template_relevance Reaxys, Template_relevance Reaxys_biocatalysis model, leveraging a vast database of chemical reactions to predict feasible synthetic routes.
One-Step Synthesis Focus: Specifically designed for one-step synthesis, it provides concise and direct routes for your target compounds, streamlining the synthesis process.
Accurate Predictions: Utilizing the extensive PISTACHIO, BKMS_METABOLIC, PISTACHIO_RINGBREAKER, REAXYS, REAXYS_BIOCATALYSIS database, our tool offers high-accuracy predictions, reflecting the latest in chemical research and data.
Strategy Settings
Precursor scoring | Relevance Heuristic |
---|---|
Min. plausibility | 0.01 |
Model | Template_relevance |
Template Set | Pistachio/Bkms_metabolic/Pistachio_ringbreaker/Reaxys/Reaxys_biocatalysis |
Top-N result to add to graph | 6 |
Feasible Synthetic Routes
Q1: How does solvation affect the conformational equilibrium of 1,1-Difluoroacetone?
A2: Theoretical calculations, particularly ab initio calculations incorporating solvation models, provide insights into the influence of solvation. Studies indicate that while calculations at the MP4/6-31G level predict only the cis conformer as a minimum energy structure in the gas phase, inclusion of solvation effects reveals a minimum for the gauche* conformation as well. [] This suggests that solvent interactions can stabilize certain conformations, leading to observable changes in the conformational equilibrium.
Q2: What unique reactivity does this compound exhibit with diazocyclopropane?
A3: Instead of the anticipated azo coupling reaction typically observed with diazonium species, this compound reacts with diazocyclopropane, generated in situ, to yield 2-(difluoromethyl)-2-methyloxaspiropentane. [] This reaction proceeds with high selectivity, targeting the carbonyl group adjacent to the difluoromethyl substituent. The resulting oxaspiropentane serves as a versatile intermediate, capable of undergoing further transformations to generate cyclobutanones or 1-vinylcyclopropanols under specific reaction conditions. []
Q3: How does the presence of fluorine atoms impact the NMR spectroscopic properties of this compound?
A4: The fluorine atoms in this compound introduce valuable coupling information in NMR spectroscopy. Studies analyzing the 1H and 13C NMR spectra, particularly the 4JHF, 1JCF, and 2JCF couplings, reveal distinct correlations between these couplings and the molecule's conformation. [, ] For instance, the 4JHF coupling constant exhibits a cos2θ dependence, where θ represents the F–C–C–C dihedral angle. Similarly, the 1JCF and 2JCF couplings display pronounced orientation dependence. This sensitivity of NMR parameters to conformational changes makes it a powerful tool for investigating the structural dynamics of this compound. []
Q4: How has computational chemistry contributed to our understanding of this compound?
A5: Computational chemistry plays a crucial role in elucidating the properties and behavior of this compound. As mentioned earlier, ab initio calculations, particularly at higher basis sets (6-311++G) or with the inclusion of solvation models, accurately predict the conformational landscape and energy differences between the cis and gauche conformers. [] These calculations provide valuable insights into the influence of electronic structure and solvent interactions on the molecule's preferred geometry. Moreover, computational studies can aid in interpreting experimental data, such as NMR coupling constants, by correlating them with calculated molecular geometries. [, ]
Disclaimer and Information on In-Vitro Research Products
Please be aware that all articles and product information presented on BenchChem are intended solely for informational purposes. The products available for purchase on BenchChem are specifically designed for in-vitro studies, which are conducted outside of living organisms. In-vitro studies, derived from the Latin term "in glass," involve experiments performed in controlled laboratory settings using cells or tissues. It is important to note that these products are not categorized as medicines or drugs, and they have not received approval from the FDA for the prevention, treatment, or cure of any medical condition, ailment, or disease. We must emphasize that any form of bodily introduction of these products into humans or animals is strictly prohibited by law. It is essential to adhere to these guidelines to ensure compliance with legal and ethical standards in research and experimentation.