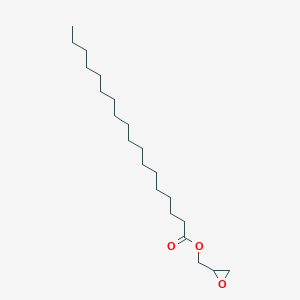
Glycidyl stearate
Overview
Description
Glycidyl stearate, also known as (oxiran-2-yl)methyl octadecanoate, is a chemical compound with the molecular formula C21H40O3 . It has an average mass of 340.540 Da and a mono-isotopic mass of 340.297760 Da . It is used as a chemical intermediate in the synthesis of glycerol, glycidyl ethers, esters, and amines .
Synthesis Analysis
Glycidyl stearate is synthesized from the epoxidation of allyl alcohol . It is also found as a by-product in the refinement of edible oils . The presence of glycidyl esters in vegetable oils is a result of research investigating the origin of 3-monochloro-1,2-propanediol (3-MCPD) and 3-MCPD esters of fatty acids in these oils .
Molecular Structure Analysis
The molecular structure of Glycidyl stearate consists of an oxirane ring (epoxide group) and a long-chain fatty acid ester . This structure contributes to its physical and chemical properties.
Physical And Chemical Properties Analysis
Glycidyl stearate is a slightly viscous liquid with a density of 0.9±0.1 g/cm³ . It has a boiling point of 424.7±18.0 °C at 760 mmHg and a flash point of 161.8±6.1 °C . It has a high LogP value of 8.73, indicating its lipophilic nature .
Scientific Research Applications
Wood Modification and Hydrophobicity Enhancement
Glycidyl stearate has been explored as a wood modifier, particularly in combination with Pinus radiata wood. Here’s how it works:
- Results :
Biomedical Applications
These applications highlight the versatility of glycidyl stearate across various fields. Researchers continue to explore its potential in novel contexts, making it an intriguing compound for scientific investigation . If you need further details or have additional questions, feel free to ask!
Safety and Hazards
Glycidyl stearate should be handled with care to avoid dust formation and inhalation of mist, gas, or vapors . Contact with skin and eyes should be avoided, and personal protective equipment, including chemical impermeable gloves, should be worn . In case of accidental release, all sources of ignition should be removed, and personnel should be evacuated to safe areas .
Future Directions
Given the potential health risks associated with Glycidyl stearate, future research should focus on developing safer and more efficient methods for its synthesis and use. Additionally, more studies are needed to fully understand its mechanism of action and the potential health effects of its consumption .
Mechanism of Action
Target of Action
Glycidyl stearate, also known as glycidyl ester of stearic acid, primarily targets a variety of fatty acids . The majority of glycidol, the parent compound of glycidyl stearate, is bound to fatty acids such as palmitate (C16:0), stearate (C18:0), oleate (C18:1), linoleate (C18:2), and linolenate (C18:3) . These fatty acids play crucial roles in various biological processes, including energy storage, cell membrane structure, and signaling pathways.
Mode of Action
Glycidyl stearate interacts with its targets through the process of esterification . When ingested as part of the diet, under the action of lipases, glycidyl esters like glycidyl stearate are believed to release glycidol . Glycidol, an epoxide, can then interact with biological molecules, potentially leading to various biochemical changes.
Biochemical Pathways
It is known that glycidyl fatty acid esters could theoretically be hydrolyzed by lipases to the parent glycidol in the gastrointestinal tract . This suggests that glycidyl stearate may influence lipid metabolism and digestion pathways.
Pharmacokinetics
It is known that glycidyl esters are formed at high temperatures during edible oil production and can be present in processed foods . This suggests that the bioavailability of glycidyl stearate may depend on dietary intake and digestive processes.
Result of Action
Glycidol, the compound released from glycidyl esters by the action of lipases, is an epoxide and has been classified as a group 2a carcinogen by iarc, indicating it is probably carcinogenic to humans . This suggests that the action of glycidyl stearate could potentially lead to harmful effects.
Action Environment
The action, efficacy, and stability of glycidyl stearate can be influenced by various environmental factors. For instance, the formation of glycidyl esters can be affected by food ingredients, cooking device, and heating time, as well as heating temperature . Therefore, the environment in which glycidyl stearate is formed and consumed can significantly impact its action.
properties
IUPAC Name |
oxiran-2-ylmethyl octadecanoate | |
---|---|---|
Source | PubChem | |
URL | https://pubchem.ncbi.nlm.nih.gov | |
Description | Data deposited in or computed by PubChem | |
InChI |
InChI=1S/C21H40O3/c1-2-3-4-5-6-7-8-9-10-11-12-13-14-15-16-17-21(22)24-19-20-18-23-20/h20H,2-19H2,1H3 | |
Source | PubChem | |
URL | https://pubchem.ncbi.nlm.nih.gov | |
Description | Data deposited in or computed by PubChem | |
InChI Key |
OUQGOXCIUOCDNN-UHFFFAOYSA-N | |
Source | PubChem | |
URL | https://pubchem.ncbi.nlm.nih.gov | |
Description | Data deposited in or computed by PubChem | |
Canonical SMILES |
CCCCCCCCCCCCCCCCCC(=O)OCC1CO1 | |
Source | PubChem | |
URL | https://pubchem.ncbi.nlm.nih.gov | |
Description | Data deposited in or computed by PubChem | |
Molecular Formula |
C21H40O3 | |
Source | PubChem | |
URL | https://pubchem.ncbi.nlm.nih.gov | |
Description | Data deposited in or computed by PubChem | |
DSSTOX Substance ID |
DTXSID601316444 | |
Record name | Glycidyl stearate | |
Source | EPA DSSTox | |
URL | https://comptox.epa.gov/dashboard/DTXSID601316444 | |
Description | DSSTox provides a high quality public chemistry resource for supporting improved predictive toxicology. | |
Molecular Weight |
340.5 g/mol | |
Source | PubChem | |
URL | https://pubchem.ncbi.nlm.nih.gov | |
Description | Data deposited in or computed by PubChem | |
CAS RN |
7460-84-6 | |
Record name | Glycidyl stearate | |
Source | CAS Common Chemistry | |
URL | https://commonchemistry.cas.org/detail?cas_rn=7460-84-6 | |
Description | CAS Common Chemistry is an open community resource for accessing chemical information. Nearly 500,000 chemical substances from CAS REGISTRY cover areas of community interest, including common and frequently regulated chemicals, and those relevant to high school and undergraduate chemistry classes. This chemical information, curated by our expert scientists, is provided in alignment with our mission as a division of the American Chemical Society. | |
Explanation | The data from CAS Common Chemistry is provided under a CC-BY-NC 4.0 license, unless otherwise stated. | |
Record name | Glycidyl stearate | |
Source | ChemIDplus | |
URL | https://pubchem.ncbi.nlm.nih.gov/substance/?source=chemidplus&sourceid=0007460846 | |
Description | ChemIDplus is a free, web search system that provides access to the structure and nomenclature authority files used for the identification of chemical substances cited in National Library of Medicine (NLM) databases, including the TOXNET system. | |
Record name | GLYCIDYL STEARATE | |
Source | DTP/NCI | |
URL | https://dtp.cancer.gov/dtpstandard/servlet/dwindex?searchtype=NSC&outputformat=html&searchlist=404228 | |
Description | The NCI Development Therapeutics Program (DTP) provides services and resources to the academic and private-sector research communities worldwide to facilitate the discovery and development of new cancer therapeutic agents. | |
Explanation | Unless otherwise indicated, all text within NCI products is free of copyright and may be reused without our permission. Credit the National Cancer Institute as the source. | |
Record name | Glycidyl stearate | |
Source | EPA DSSTox | |
URL | https://comptox.epa.gov/dashboard/DTXSID601316444 | |
Description | DSSTox provides a high quality public chemistry resource for supporting improved predictive toxicology. | |
Record name | GLYCIDYL STEARATE | |
Source | FDA Global Substance Registration System (GSRS) | |
URL | https://gsrs.ncats.nih.gov/ginas/app/beta/substances/Q7QG7S8K60 | |
Description | The FDA Global Substance Registration System (GSRS) enables the efficient and accurate exchange of information on what substances are in regulated products. Instead of relying on names, which vary across regulatory domains, countries, and regions, the GSRS knowledge base makes it possible for substances to be defined by standardized, scientific descriptions. | |
Explanation | Unless otherwise noted, the contents of the FDA website (www.fda.gov), both text and graphics, are not copyrighted. They are in the public domain and may be republished, reprinted and otherwise used freely by anyone without the need to obtain permission from FDA. Credit to the U.S. Food and Drug Administration as the source is appreciated but not required. | |
Retrosynthesis Analysis
AI-Powered Synthesis Planning: Our tool employs the Template_relevance Pistachio, Template_relevance Bkms_metabolic, Template_relevance Pistachio_ringbreaker, Template_relevance Reaxys, Template_relevance Reaxys_biocatalysis model, leveraging a vast database of chemical reactions to predict feasible synthetic routes.
One-Step Synthesis Focus: Specifically designed for one-step synthesis, it provides concise and direct routes for your target compounds, streamlining the synthesis process.
Accurate Predictions: Utilizing the extensive PISTACHIO, BKMS_METABOLIC, PISTACHIO_RINGBREAKER, REAXYS, REAXYS_BIOCATALYSIS database, our tool offers high-accuracy predictions, reflecting the latest in chemical research and data.
Strategy Settings
Precursor scoring | Relevance Heuristic |
---|---|
Min. plausibility | 0.01 |
Model | Template_relevance |
Template Set | Pistachio/Bkms_metabolic/Pistachio_ringbreaker/Reaxys/Reaxys_biocatalysis |
Top-N result to add to graph | 6 |
Feasible Synthetic Routes
Q & A
Q1: What are the main applications of Glycidyl stearate?
A1: Glycidyl stearate is primarily utilized as a hydrophobic finishing agent for materials like cotton fabrics. [] This compound enhances the material's resistance to water absorption, increasing its durability. Additionally, research indicates its potential as a diagnostic marker for prostate cancer, particularly in cases where the prostate-specific antigen (PSA) levels fall within a diagnostically ambiguous range. []
Q2: How does Glycidyl stearate contribute to hydrophobicity?
A2: When applied to cotton fabrics, Glycidyl stearate chemically interacts with the cellulose fibers. [] This interaction is evidenced by FTIR, SEM, and EDX analyses. This treatment results in a significant increase in the water contact angle, reaching up to 152 degrees, and a prolonged water drop absorption time, reaching 200 minutes under optimal conditions. []
Q3: What factors influence the effectiveness of Glycidyl stearate in hydrophobic treatments?
A3: Research suggests that factors such as the concentration of Glycidyl stearate used, the temperature, and the duration of the curing treatment can significantly influence its effectiveness in imparting hydrophobicity. [] Additionally, the presence of other chemicals, like NaOH, during the treatment process can also play a role. []
Q4: Beyond hydrophobic treatments, are there other areas where Glycidyl stearate is being investigated?
A4: Yes, recent research has explored the potential of using Glycidyl stearate as a component in biodegradable cationic surfactants. [] These types of surfactants are of interest due to their potential in various applications while being more environmentally friendly.
Q5: What is the significance of Glycidyl stearate in metabolomics studies?
A5: Glycidyl stearate has been identified as a potential biomarker in metabolomics studies, specifically in the context of distinguishing prostate cancer from benign prostatic hyperplasia (BPH) within the PSA gray zone. [] Its presence in serum, alongside other lipid metabolites, showed a correlation with PCa diagnosis. []
Q6: Are there any potential concerns regarding Glycidyl stearate in food products?
A6: While not directly addressed in the provided abstracts, Glycidyl stearate is structurally similar to glycidyl fatty acid esters, which are a concern in edible oils. [, ] These esters can potentially form during the refining process and have been linked to health concerns. Further research would be needed to assess if similar concerns exist for Glycidyl stearate in food applications.
Q7: What analytical techniques are used to study Glycidyl stearate?
A7: Various analytical techniques have been employed to study Glycidyl stearate. For instance, in its application for hydrophobic treatments, FTIR, SEM, and EDX analyses were used to confirm its presence and interaction with the treated material. [] In metabolomics studies, techniques like LC-MS/MS and NMR are used for identification and quantification of Glycidyl stearate and other metabolites. [, ]
Disclaimer and Information on In-Vitro Research Products
Please be aware that all articles and product information presented on BenchChem are intended solely for informational purposes. The products available for purchase on BenchChem are specifically designed for in-vitro studies, which are conducted outside of living organisms. In-vitro studies, derived from the Latin term "in glass," involve experiments performed in controlled laboratory settings using cells or tissues. It is important to note that these products are not categorized as medicines or drugs, and they have not received approval from the FDA for the prevention, treatment, or cure of any medical condition, ailment, or disease. We must emphasize that any form of bodily introduction of these products into humans or animals is strictly prohibited by law. It is essential to adhere to these guidelines to ensure compliance with legal and ethical standards in research and experimentation.