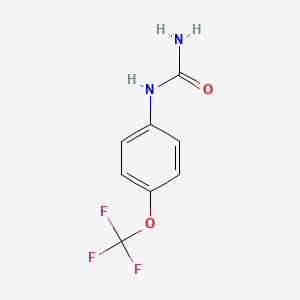
(4-(Trifluoromethoxy)phenyl)urea
Overview
Description
(4-(Trifluoromethoxy)phenyl)urea is an organic compound characterized by the presence of a trifluoromethoxy group attached to a phenyl ring, which is further connected to a urea moiety
Mechanism of Action
Target of Action
The primary target of (4-(Trifluoromethoxy)phenyl)urea is the human soluble epoxide hydrolase (sEH) enzyme . This enzyme plays a crucial role in the metabolism of epoxyeicosatrienoic acids (EETs), which are signaling molecules involved in various physiological processes, including inflammation and blood pressure regulation .
Mode of Action
This compound acts as an inhibitor of sEH . It binds to the active site of the enzyme, preventing it from metabolizing its substrates .
Biochemical Pathways
The inhibition of sEH affects the metabolic pathway of EETs . Under normal conditions, sEH metabolizes EETs into less active dihydroxyeicosatrienoic acids (DHETs). When seh is inhibited by this compound, eets accumulate, leading to enhanced signaling through their respective pathways .
Pharmacokinetics
It is known that the compound is a small molecule, with a molecular weight of 2202 , suggesting that it may have good bioavailability
Result of Action
The inhibition of sEH by this compound leads to an increase in the levels of EETs . This can result in various cellular effects, depending on the specific roles of EETs in different cell types. For example, increased EET levels can lead to vasodilation, anti-inflammatory effects, and protection of the heart during ischemia .
Biochemical Analysis
Biochemical Properties
(4-(Trifluoromethoxy)phenyl)urea plays a crucial role in biochemical reactions, primarily as an inhibitor of soluble epoxide hydrolase. This enzyme is involved in the metabolism of epoxides to diols, which are less reactive and more water-soluble. By inhibiting soluble epoxide hydrolase, this compound can modulate the levels of epoxides and diols in the body, impacting various physiological processes. The compound interacts with the active site of soluble epoxide hydrolase, forming hydrogen bonds and other interactions that stabilize the inhibitor-enzyme complex . Additionally, this compound has been shown to interact with other biomolecules, such as proteins involved in inflammation and pain pathways .
Cellular Effects
The effects of this compound on various cell types and cellular processes are profound. This compound influences cell function by modulating cell signaling pathways, gene expression, and cellular metabolism. For instance, by inhibiting soluble epoxide hydrolase, this compound can reduce inflammation and pain by altering the levels of epoxyeicosatrienoic acids, which are signaling molecules involved in these processes . Furthermore, this compound has been shown to affect the proliferation and migration of endothelial cells, which are critical for angiogenesis .
Molecular Mechanism
The molecular mechanism of action of this compound involves its binding interactions with soluble epoxide hydrolase and other biomolecules. The compound binds to the active site of soluble epoxide hydrolase, forming hydrogen bonds and hydrophobic interactions that inhibit the enzyme’s activity . This inhibition leads to an accumulation of epoxyeicosatrienoic acids, which have various biological effects, including anti-inflammatory and vasodilatory actions . Additionally, this compound may influence gene expression by modulating the activity of transcription factors and other regulatory proteins .
Temporal Effects in Laboratory Settings
In laboratory settings, the effects of this compound can change over time due to its stability, degradation, and long-term impact on cellular function. Studies have shown that this compound is relatively stable under standard laboratory conditions, but it may degrade over extended periods or under specific conditions . Long-term exposure to this compound in in vitro and in vivo studies has revealed sustained inhibition of soluble epoxide hydrolase and prolonged anti-inflammatory effects .
Dosage Effects in Animal Models
The effects of this compound vary with different dosages in animal models. At lower doses, the compound effectively inhibits soluble epoxide hydrolase and exerts anti-inflammatory and analgesic effects without significant toxicity . At higher doses, this compound may cause adverse effects, such as hepatotoxicity and nephrotoxicity . These threshold effects highlight the importance of dose optimization in therapeutic applications.
Metabolic Pathways
This compound is involved in several metabolic pathways, primarily through its interaction with soluble epoxide hydrolase. The compound is metabolized via oxidation and amide hydrolysis, leading to the formation of various metabolites . These metabolites can further interact with enzymes and cofactors, influencing metabolic flux and metabolite levels in the body . Understanding the metabolic pathways of this compound is crucial for assessing its safety and efficacy in therapeutic applications.
Transport and Distribution
The transport and distribution of this compound within cells and tissues are influenced by its lipophilic nature and interactions with transporters and binding proteins. The compound can readily cross cell membranes and accumulate in lipid-rich tissues . Additionally, this compound may interact with specific transporters that facilitate its uptake and distribution within the body . These interactions can affect the localization and accumulation of the compound, impacting its biological activity.
Subcellular Localization
The subcellular localization of this compound is critical for its activity and function. The compound is primarily localized in the cytoplasm, where it interacts with soluble epoxide hydrolase and other cytoplasmic proteins . Additionally, this compound may be targeted to specific subcellular compartments or organelles through post-translational modifications or targeting signals . These localization patterns can influence the compound’s efficacy and specificity in modulating cellular processes.
Preparation Methods
Synthetic Routes and Reaction Conditions
The synthesis of (4-(trifluoromethoxy)phenyl)urea typically involves the reaction of 4-(trifluoromethoxy)aniline with an isocyanate derivative. One common method is the reaction of 4-(trifluoromethoxy)phenyl isocyanate with aliphatic diamines, yielding N,N′-disubstituted bis-ureas containing the 4-(trifluoromethoxy)phenyl fragment . This reaction is usually carried out in an organic solvent such as dichloromethane or toluene, under mild conditions, and can achieve yields ranging from 58% to 80% .
Industrial Production Methods
In an industrial setting, the production of this compound may involve similar synthetic routes but on a larger scale. The process would be optimized for higher efficiency and yield, potentially incorporating continuous flow reactors and automated systems to ensure consistent quality and scalability.
Chemical Reactions Analysis
Types of Reactions
(4-(Trifluoromethoxy)phenyl)urea can undergo various chemical reactions, including:
Substitution Reactions: The trifluoromethoxy group can participate in nucleophilic aromatic substitution reactions, where nucleophiles replace the trifluoromethoxy group under appropriate conditions.
Oxidation and Reduction: The phenyl ring and urea moiety can undergo oxidation and reduction reactions, respectively, altering the oxidation state of the compound.
Hydrolysis: The urea group can be hydrolyzed under acidic or basic conditions, leading to the formation of corresponding amines and carbon dioxide.
Common Reagents and Conditions
Substitution Reactions: Common reagents include nucleophiles such as amines or thiols, often in the presence of a base like sodium hydride or potassium carbonate.
Oxidation: Oxidizing agents such as potassium permanganate or chromium trioxide can be used.
Reduction: Reducing agents like lithium aluminum hydride or sodium borohydride are typically employed.
Hydrolysis: Acidic conditions (e.g., hydrochloric acid) or basic conditions (e.g., sodium hydroxide) are used for hydrolysis reactions.
Major Products Formed
The major products formed from these reactions depend on the specific conditions and reagents used. For example, nucleophilic substitution can yield various substituted phenylureas, while hydrolysis results in the formation of amines and carbon dioxide.
Scientific Research Applications
Chemistry
In chemistry, (4-(trifluoromethoxy)phenyl)urea is used as a building block for synthesizing more complex molecules. Its unique trifluoromethoxy group imparts lipophilicity and electron-withdrawing properties, making it valuable in designing molecules with specific electronic characteristics.
Biology and Medicine
In medicinal chemistry, this compound derivatives have shown promise as inhibitors of human soluble epoxide hydrolase (sEH), an enzyme involved in the metabolism of fatty acid epoxides . These inhibitors have potential therapeutic applications in treating conditions such as hypertension, inflammation, and certain cancers .
Industry
In the industrial sector, this compound and its derivatives are explored for their potential use in agrochemicals, such as insecticides and herbicides. Their ability to interact with biological targets makes them suitable for developing new pest control agents .
Comparison with Similar Compounds
Similar Compounds
(4-Methoxyphenyl)urea: Similar in structure but lacks the trifluoromethoxy group, resulting in different electronic properties and biological activity.
(4-Trifluoromethylphenyl)urea: Contains a trifluoromethyl group instead of a trifluoromethoxy group, affecting its lipophilicity and reactivity.
(4-Chlorophenyl)urea: Substitutes a chlorine atom for the trifluoromethoxy group, leading to variations in its chemical and biological properties.
Uniqueness
The presence of the trifluoromethoxy group in (4-(trifluoromethoxy)phenyl)urea imparts unique properties, such as increased lipophilicity and electron-withdrawing effects, which can enhance its binding affinity to biological targets and improve its pharmacokinetic profile. These characteristics make it a valuable compound in the development of new therapeutic agents and industrial applications.
Properties
IUPAC Name |
[4-(trifluoromethoxy)phenyl]urea | |
---|---|---|
Source | PubChem | |
URL | https://pubchem.ncbi.nlm.nih.gov | |
Description | Data deposited in or computed by PubChem | |
InChI |
InChI=1S/C8H7F3N2O2/c9-8(10,11)15-6-3-1-5(2-4-6)13-7(12)14/h1-4H,(H3,12,13,14) | |
Source | PubChem | |
URL | https://pubchem.ncbi.nlm.nih.gov | |
Description | Data deposited in or computed by PubChem | |
InChI Key |
LOSFVIMHTOMZKT-UHFFFAOYSA-N | |
Source | PubChem | |
URL | https://pubchem.ncbi.nlm.nih.gov | |
Description | Data deposited in or computed by PubChem | |
Canonical SMILES |
C1=CC(=CC=C1NC(=O)N)OC(F)(F)F | |
Source | PubChem | |
URL | https://pubchem.ncbi.nlm.nih.gov | |
Description | Data deposited in or computed by PubChem | |
Molecular Formula |
C8H7F3N2O2 | |
Source | PubChem | |
URL | https://pubchem.ncbi.nlm.nih.gov | |
Description | Data deposited in or computed by PubChem | |
DSSTOX Substance ID |
DTXSID50896798 | |
Record name | (4-(Trifluoromethoxy)phenyl)urea | |
Source | EPA DSSTox | |
URL | https://comptox.epa.gov/dashboard/DTXSID50896798 | |
Description | DSSTox provides a high quality public chemistry resource for supporting improved predictive toxicology. | |
Molecular Weight |
220.15 g/mol | |
Source | PubChem | |
URL | https://pubchem.ncbi.nlm.nih.gov | |
Description | Data deposited in or computed by PubChem | |
CAS No. |
82971-90-2 | |
Record name | (4-(Trifluoromethoxy)phenyl)urea | |
Source | ChemIDplus | |
URL | https://pubchem.ncbi.nlm.nih.gov/substance/?source=chemidplus&sourceid=0082971902 | |
Description | ChemIDplus is a free, web search system that provides access to the structure and nomenclature authority files used for the identification of chemical substances cited in National Library of Medicine (NLM) databases, including the TOXNET system. | |
Record name | (4-(trifluoromethoxy)phenyl)urea | |
Source | DrugBank | |
URL | https://www.drugbank.ca/drugs/DB15610 | |
Description | The DrugBank database is a unique bioinformatics and cheminformatics resource that combines detailed drug (i.e. chemical, pharmacological and pharmaceutical) data with comprehensive drug target (i.e. sequence, structure, and pathway) information. | |
Explanation | Creative Common's Attribution-NonCommercial 4.0 International License (http://creativecommons.org/licenses/by-nc/4.0/legalcode) | |
Record name | (4-(Trifluoromethoxy)phenyl)urea | |
Source | EPA DSSTox | |
URL | https://comptox.epa.gov/dashboard/DTXSID50896798 | |
Description | DSSTox provides a high quality public chemistry resource for supporting improved predictive toxicology. | |
Record name | (4-(TRIFLUOROMETHOXY)PHENYL)UREA | |
Source | FDA Global Substance Registration System (GSRS) | |
URL | https://gsrs.ncats.nih.gov/ginas/app/beta/substances/SQP4E8O29W | |
Description | The FDA Global Substance Registration System (GSRS) enables the efficient and accurate exchange of information on what substances are in regulated products. Instead of relying on names, which vary across regulatory domains, countries, and regions, the GSRS knowledge base makes it possible for substances to be defined by standardized, scientific descriptions. | |
Explanation | Unless otherwise noted, the contents of the FDA website (www.fda.gov), both text and graphics, are not copyrighted. They are in the public domain and may be republished, reprinted and otherwise used freely by anyone without the need to obtain permission from FDA. Credit to the U.S. Food and Drug Administration as the source is appreciated but not required. | |
Retrosynthesis Analysis
AI-Powered Synthesis Planning: Our tool employs the Template_relevance Pistachio, Template_relevance Bkms_metabolic, Template_relevance Pistachio_ringbreaker, Template_relevance Reaxys, Template_relevance Reaxys_biocatalysis model, leveraging a vast database of chemical reactions to predict feasible synthetic routes.
One-Step Synthesis Focus: Specifically designed for one-step synthesis, it provides concise and direct routes for your target compounds, streamlining the synthesis process.
Accurate Predictions: Utilizing the extensive PISTACHIO, BKMS_METABOLIC, PISTACHIO_RINGBREAKER, REAXYS, REAXYS_BIOCATALYSIS database, our tool offers high-accuracy predictions, reflecting the latest in chemical research and data.
Strategy Settings
Precursor scoring | Relevance Heuristic |
---|---|
Min. plausibility | 0.01 |
Model | Template_relevance |
Template Set | Pistachio/Bkms_metabolic/Pistachio_ringbreaker/Reaxys/Reaxys_biocatalysis |
Top-N result to add to graph | 6 |
Feasible Synthetic Routes
Q1: What enzymes are known to be inhibited by compounds containing the (4-(trifluoromethoxy)phenyl)urea moiety?
A1: Research indicates that compounds containing the this compound moiety exhibit potent inhibition of soluble epoxide hydrolase (sEH) [, , , , , , , , , ]. Additionally, some derivatives have shown inhibitory activity against P2Y1 receptors [, ] and p38β kinase [].
Q2: What are the downstream effects of sEH inhibition by this compound derivatives?
A2: Inhibiting sEH with this compound derivatives leads to the stabilization of epoxyeicosatrienoic acids (EETs) [, , ]. EETs possess various beneficial effects, including anti-inflammatory, analgesic, and neuroprotective properties [, , , , ].
Q3: How does the inhibition of sEH by this compound containing compounds translate to potential therapeutic benefits?
A3: Preclinical studies suggest that sEH inhibition by this compound derivatives may hold therapeutic potential for various conditions, including:
- Pain management: These compounds have shown efficacy in reducing inflammatory and neuropathic pain in animal models [, , , ].
- Cardiovascular diseases: Studies have demonstrated their potential in alleviating hypertension, cardiac hypertrophy, and ischemia-reperfusion injury [, , , ].
- Neurodegenerative disorders: Research suggests possible benefits in models of Alzheimer's disease by reducing tau hyperphosphorylation and amyloid toxicity [, , ].
- Pulmonary hypertension: Studies indicate that sEH inhibition might not worsen or contribute to the development of pulmonary hypertension [].
Q4: How do structural modifications to the this compound scaffold influence its activity against sEH?
A4: Studies have demonstrated that modifications to the acyl group attached to the piperidine ring of 1-aryl-3-(1-acylpiperidin-4-yl)urea derivatives can significantly impact pharmacokinetic properties and potency against sEH []. For instance, replacing the adamantyl group with a cyclopropyl group led to a significant improvement in potency, Cmax, and AUC [].
Q5: Are there structural modifications that enhance the selectivity of this compound derivatives for specific targets?
A5: While this compound derivatives display promising activity against sEH, research indicates that they can also interact with other targets like P2Y1 receptors [, ]. Structural modifications are crucial for achieving greater target selectivity. For example, incorporating specific bulky substituents into the molecule has led to the development of compounds with improved selectivity for P2Y1 receptors over sEH [, ].
Q6: How does the TMDD of this compound sEH inhibitors affect their dosing and efficacy?
A6: The TMDD observed with these inhibitors adds complexity to their pharmacokinetic profiles [, ]. Understanding the extent of target binding and its impact on drug exposure is crucial for optimizing dosing regimens and achieving sustained therapeutic efficacy.
Q7: Are there specific transporters involved in the distribution and elimination of this compound compounds?
A7: While specific transporters haven't been extensively characterized in the provided research, it is highly likely that drug transporters play a role in the absorption, distribution, and elimination of these compounds, as is common for many small molecules. Further research is necessary to elucidate the specific transporters involved.
Q8: What analytical techniques are commonly employed to characterize and quantify this compound compounds?
A8: Researchers utilize a combination of techniques for the analysis of these compounds. Liquid chromatography coupled with tandem mass spectrometry (LC-MS/MS) is frequently used to identify and quantify these compounds and their metabolites in biological matrices [, ].
Disclaimer and Information on In-Vitro Research Products
Please be aware that all articles and product information presented on BenchChem are intended solely for informational purposes. The products available for purchase on BenchChem are specifically designed for in-vitro studies, which are conducted outside of living organisms. In-vitro studies, derived from the Latin term "in glass," involve experiments performed in controlled laboratory settings using cells or tissues. It is important to note that these products are not categorized as medicines or drugs, and they have not received approval from the FDA for the prevention, treatment, or cure of any medical condition, ailment, or disease. We must emphasize that any form of bodily introduction of these products into humans or animals is strictly prohibited by law. It is essential to adhere to these guidelines to ensure compliance with legal and ethical standards in research and experimentation.