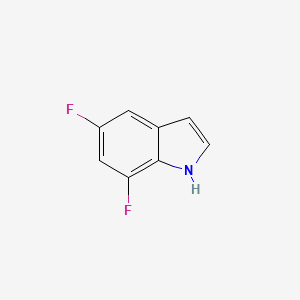
5,7-Difluoroindole
Overview
Description
5,7-Difluoroindole is a useful research compound. Its molecular formula is C8H5F2N and its molecular weight is 153.13 g/mol. The purity is usually 95%.
The exact mass of the compound this compound is unknown and the complexity rating of the compound is unknown. The United Nations designated GHS hazard class pictogram is Irritant, and the GHS signal word is WarningThe storage condition is unknown. Please store according to label instructions upon receipt of goods.
BenchChem offers high-quality this compound suitable for many research applications. Different packaging options are available to accommodate customers' requirements. Please inquire for more information about this compound including the price, delivery time, and more detailed information at info@benchchem.com.
Biochemical Analysis
Biochemical Properties
5,7-Difluoroindole plays a crucial role in various biochemical reactions. It has been identified as a potent and metabolically stable influenza inhibitor . The compound interacts with several enzymes and proteins, including the influenza PB2 cap-binding region, where it binds effectively to inhibit viral replication . Additionally, this compound is not at risk of metabolism via aldehyde oxidase, which is an advantage over other inhibitors in its class .
Cellular Effects
This compound has been shown to influence various cellular processes. It inhibits biofilm formation and blood hemolysis in Pseudomonas aeruginosa without affecting the growth of planktonic cells . This compound also reduces the production of quorum-sensing-regulated virulence factors, such as pyocyanin and rhamnolipid, and suppresses swarming motility and protease activity . These effects suggest that this compound can modulate cell signaling pathways and gene expression in bacterial cells.
Molecular Mechanism
The molecular mechanism of this compound involves its binding interactions with specific biomolecules. For instance, it binds to the influenza PB2 cap-binding region, inhibiting the viral polymerase activity and preventing viral replication . This binding interaction is crucial for its antiviral activity. Additionally, this compound’s ability to inhibit biofilm formation and virulence factor production in Pseudomonas aeruginosa is likely due to its interference with quorum-sensing pathways .
Temporal Effects in Laboratory Settings
In laboratory settings, the effects of this compound have been observed to change over time. The compound demonstrates stability and retains its inhibitory activity against influenza over extended periods
Dosage Effects in Animal Models
The effects of this compound vary with different dosages in animal models. At lower doses, the compound exhibits potent antiviral activity without significant toxicity At higher doses, there may be potential adverse effects, although specific toxicological data for this compound in animal models are limited
Metabolic Pathways
This compound is involved in metabolic pathways that include interactions with various enzymes and cofactors. The compound’s metabolic stability is enhanced by its resistance to aldehyde oxidase metabolism . This stability allows it to maintain its biological activity and effectiveness in inhibiting viral replication and bacterial virulence.
Properties
IUPAC Name |
5,7-difluoro-1H-indole | |
---|---|---|
Source | PubChem | |
URL | https://pubchem.ncbi.nlm.nih.gov | |
Description | Data deposited in or computed by PubChem | |
InChI |
InChI=1S/C8H5F2N/c9-6-3-5-1-2-11-8(5)7(10)4-6/h1-4,11H | |
Source | PubChem | |
URL | https://pubchem.ncbi.nlm.nih.gov | |
Description | Data deposited in or computed by PubChem | |
InChI Key |
WZPOGQRJXZGSMH-UHFFFAOYSA-N | |
Source | PubChem | |
URL | https://pubchem.ncbi.nlm.nih.gov | |
Description | Data deposited in or computed by PubChem | |
Canonical SMILES |
C1=CNC2=C(C=C(C=C21)F)F | |
Source | PubChem | |
URL | https://pubchem.ncbi.nlm.nih.gov | |
Description | Data deposited in or computed by PubChem | |
Molecular Formula |
C8H5F2N | |
Source | PubChem | |
URL | https://pubchem.ncbi.nlm.nih.gov | |
Description | Data deposited in or computed by PubChem | |
DSSTOX Substance ID |
DTXSID90381097 | |
Record name | 5,7-Difluoroindole | |
Source | EPA DSSTox | |
URL | https://comptox.epa.gov/dashboard/DTXSID90381097 | |
Description | DSSTox provides a high quality public chemistry resource for supporting improved predictive toxicology. | |
Molecular Weight |
153.13 g/mol | |
Source | PubChem | |
URL | https://pubchem.ncbi.nlm.nih.gov | |
Description | Data deposited in or computed by PubChem | |
CAS No. |
301856-25-7 | |
Record name | 5,7-Difluoroindole | |
Source | EPA DSSTox | |
URL | https://comptox.epa.gov/dashboard/DTXSID90381097 | |
Description | DSSTox provides a high quality public chemistry resource for supporting improved predictive toxicology. | |
Record name | 5,7-difluoro-1H-indole | |
Source | European Chemicals Agency (ECHA) | |
URL | https://echa.europa.eu/information-on-chemicals | |
Description | The European Chemicals Agency (ECHA) is an agency of the European Union which is the driving force among regulatory authorities in implementing the EU's groundbreaking chemicals legislation for the benefit of human health and the environment as well as for innovation and competitiveness. | |
Explanation | Use of the information, documents and data from the ECHA website is subject to the terms and conditions of this Legal Notice, and subject to other binding limitations provided for under applicable law, the information, documents and data made available on the ECHA website may be reproduced, distributed and/or used, totally or in part, for non-commercial purposes provided that ECHA is acknowledged as the source: "Source: European Chemicals Agency, http://echa.europa.eu/". Such acknowledgement must be included in each copy of the material. ECHA permits and encourages organisations and individuals to create links to the ECHA website under the following cumulative conditions: Links can only be made to webpages that provide a link to the Legal Notice page. | |
Synthesis routes and methods I
Procedure details
Synthesis routes and methods II
Procedure details
Retrosynthesis Analysis
AI-Powered Synthesis Planning: Our tool employs the Template_relevance Pistachio, Template_relevance Bkms_metabolic, Template_relevance Pistachio_ringbreaker, Template_relevance Reaxys, Template_relevance Reaxys_biocatalysis model, leveraging a vast database of chemical reactions to predict feasible synthetic routes.
One-Step Synthesis Focus: Specifically designed for one-step synthesis, it provides concise and direct routes for your target compounds, streamlining the synthesis process.
Accurate Predictions: Utilizing the extensive PISTACHIO, BKMS_METABOLIC, PISTACHIO_RINGBREAKER, REAXYS, REAXYS_BIOCATALYSIS database, our tool offers high-accuracy predictions, reflecting the latest in chemical research and data.
Strategy Settings
Precursor scoring | Relevance Heuristic |
---|---|
Min. plausibility | 0.01 |
Model | Template_relevance |
Template Set | Pistachio/Bkms_metabolic/Pistachio_ringbreaker/Reaxys/Reaxys_biocatalysis |
Top-N result to add to graph | 6 |
Feasible Synthetic Routes
Q1: How does 5,7-difluoroindole interact with its target and what are the downstream effects?
A1: this compound (specifically, derivative 11a in the study) targets the PB2 subunit of the influenza virus polymerase. It binds to the cap-binding region of PB2 []. This interaction disrupts the virus's ability to "cap-snatch," a process crucial for the initiation of viral mRNA synthesis. By inhibiting this process, this compound ultimately hinders the replication of the influenza virus. The crystal structure of 11a bound to influenza A PB2 cap region is available in the Protein Data Bank (PDB) [].
Q2: What is known about the structure-activity relationship (SAR) of this compound derivatives as influenza inhibitors?
A2: The research focused on exploring 7-fluoro-substituted indoles as potential bioisosteres for the 7-azaindole scaffold found in Pimodivir, a known PB2 inhibitor. The study highlights that incorporating a fluorine atom at the 5-position of the indole ring, alongside the 7-fluoro substitution, significantly enhanced the compound's potency and metabolic stability []. This suggests that the presence and position of fluorine atoms play a crucial role in the compound's interaction with the PB2 target and its overall efficacy as an influenza inhibitor.
Disclaimer and Information on In-Vitro Research Products
Please be aware that all articles and product information presented on BenchChem are intended solely for informational purposes. The products available for purchase on BenchChem are specifically designed for in-vitro studies, which are conducted outside of living organisms. In-vitro studies, derived from the Latin term "in glass," involve experiments performed in controlled laboratory settings using cells or tissues. It is important to note that these products are not categorized as medicines or drugs, and they have not received approval from the FDA for the prevention, treatment, or cure of any medical condition, ailment, or disease. We must emphasize that any form of bodily introduction of these products into humans or animals is strictly prohibited by law. It is essential to adhere to these guidelines to ensure compliance with legal and ethical standards in research and experimentation.