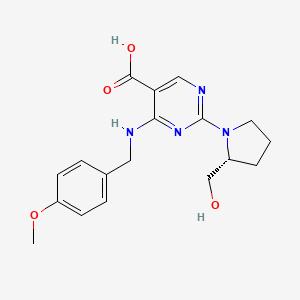
Avanafil impurity 24
- Click on QUICK INQUIRY to receive a quote from our team of experts.
- With the quality product at a COMPETITIVE price, you can focus more on your research.
Overview
Description
Avanafil impurity 24 is a process-related impurity found in the synthesis of Avanafil, a phosphodiesterase type 5 inhibitor used to treat erectile dysfunction in men . The identification and characterization of such impurities are crucial for ensuring the safety and efficacy of pharmaceutical products.
Preparation Methods
The synthesis of Avanafil impurity 24 involves several steps, including the use of specific reagents and conditions. The synthetic route typically involves the use of 1-ethyl-(3-dimethylaminopropyl)carbamyldiimide hydrochloride and 1-hydroxybenzotriazole as reactive acid-amine binding agents . These reagents facilitate the formation of the impurity during the synthesis process. The reaction conditions, such as temperature and solvent choice, play a significant role in the formation and isolation of the impurity.
Chemical Reactions Analysis
Avanafil impurity 24 undergoes various chemical reactions, including oxidation, reduction, and substitution reactions. Common reagents used in these reactions include ammonium formate and acetonitrile . The major products formed from these reactions depend on the specific conditions and reagents used. For example, the use of ammonium formate and acetonitrile in ultra-high performance liquid chromatography (UPLC) allows for the efficient separation and identification of the impurity .
Scientific Research Applications
Avanafil impurity 24 has several scientific research applications. It is used in the development and validation of analytical methods, such as UPLC, for the quality control of Avanafil . The impurity is also studied to understand its impact on the safety and efficacy of the final pharmaceutical product. Additionally, research on this compound contributes to the broader understanding of process-related impurities in drug synthesis and their potential effects on human health.
Mechanism of Action
The mechanism of action of Avanafil impurity 24 is not well-documented, as it is primarily a by-product of the synthesis process. its presence in the final pharmaceutical product can affect the overall efficacy and safety of Avanafil. The impurity may interact with molecular targets and pathways involved in the pharmacological action of Avanafil, potentially leading to adverse effects.
Comparison with Similar Compounds
Avanafil impurity 24 can be compared with other process-related impurities found in the synthesis of phosphodiesterase type 5 inhibitors. Similar compounds include Imp-A, Imp-B, Imp-C, and Imp-D, which are also identified and characterized during the synthesis of Avanafil . Each impurity has unique structural and chemical properties that distinguish it from others. The identification and characterization of these impurities are essential for ensuring the quality and safety of the final pharmaceutical product.
Biological Activity
Avanafil impurity 24 is one of the process-related impurities associated with avanafil, a phosphodiesterase type 5 (PDE5) inhibitor used primarily for treating erectile dysfunction. Understanding the biological activity of this impurity is crucial for assessing the safety and efficacy of avanafil formulations. This article reviews the synthesis, characterization, and biological implications of this compound based on recent research findings.
Overview of Avanafil and Its Impurities
Avanafil's primary mechanism involves inhibiting PDE5, which leads to increased levels of cyclic guanosine monophosphate (cGMP) in the corpus cavernosum, facilitating erection upon sexual stimulation. The presence of impurities like impurity 24 can potentially affect both the pharmacological activity and safety profile of the drug.
Identification and Synthesis
Recent studies have identified various impurities in avanafil, including impurity 24. The identification process typically involves sophisticated techniques such as ultra-high-performance liquid chromatography (UPLC) coupled with mass spectrometry (MS) .
Table 1: Summary of Avanafil Impurities
Impurity Name | Retention Time (min) | Content Range (%) |
---|---|---|
Impurity A | 4.29 | 0.29 - 1.63 |
Impurity B | 5.02 | 0.29 - 1.63 |
Impurity C | 6.02 | 0.29 - 1.63 |
Impurity D | 7.85 | 0.29 - 1.63 |
Impurity 24 | TBD | TBD |
Biological Activity
The biological activity of this compound has not been extensively characterized in literature; however, its structural similarities to other known PDE5 inhibitors suggest potential activity in modulating cGMP levels.
As with other PDE5 inhibitors, it is hypothesized that impurity 24 may act by inhibiting the PDE5 enzyme, thereby increasing cGMP concentration in smooth muscle cells. This action could lead to enhanced vasodilation and increased blood flow to erectile tissues.
Stability Studies
Stability studies have shown that avanafil can degrade under various stress conditions, which may also affect its impurities . The degradation products can include different impurities that might exhibit varying biological activities.
Table 2: Stability-Indicating HPLC Method Results
Condition | Observed Degradants | Major Impurities Detected |
---|---|---|
Acidic Stress | Acid impurity | Deschloro impurity |
Oxidative Stress | Unknown impurity | Dichloro impurity |
Thermal Stress | Unknown impurities | Dimer impurity |
Humidity Stress | Unknown impurities | Diamine impurity |
Properties
Molecular Formula |
C18H22N4O4 |
---|---|
Molecular Weight |
358.4 g/mol |
IUPAC Name |
2-[(2R)-2-(hydroxymethyl)pyrrolidin-1-yl]-4-[(4-methoxyphenyl)methylamino]pyrimidine-5-carboxylic acid |
InChI |
InChI=1S/C18H22N4O4/c1-26-14-6-4-12(5-7-14)9-19-16-15(17(24)25)10-20-18(21-16)22-8-2-3-13(22)11-23/h4-7,10,13,23H,2-3,8-9,11H2,1H3,(H,24,25)(H,19,20,21)/t13-/m1/s1 |
InChI Key |
QOBLCWBBKFBHQF-CYBMUJFWSA-N |
Isomeric SMILES |
COC1=CC=C(C=C1)CNC2=NC(=NC=C2C(=O)O)N3CCC[C@@H]3CO |
Canonical SMILES |
COC1=CC=C(C=C1)CNC2=NC(=NC=C2C(=O)O)N3CCCC3CO |
Origin of Product |
United States |
Disclaimer and Information on In-Vitro Research Products
Please be aware that all articles and product information presented on BenchChem are intended solely for informational purposes. The products available for purchase on BenchChem are specifically designed for in-vitro studies, which are conducted outside of living organisms. In-vitro studies, derived from the Latin term "in glass," involve experiments performed in controlled laboratory settings using cells or tissues. It is important to note that these products are not categorized as medicines or drugs, and they have not received approval from the FDA for the prevention, treatment, or cure of any medical condition, ailment, or disease. We must emphasize that any form of bodily introduction of these products into humans or animals is strictly prohibited by law. It is essential to adhere to these guidelines to ensure compliance with legal and ethical standards in research and experimentation.