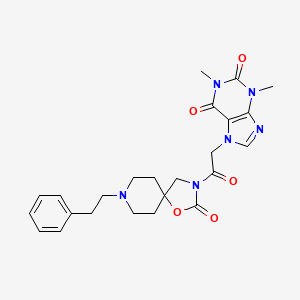
Spirofylline
Overview
Description
Spirofylline is a chemical compound known for its potential as a bronchodilator, which means it can help relax and open the air passages in the lungs, making it easier to breathe. This compound has shown promise in the treatment of respiratory conditions such as asthma, bronchitis, and emphysema .
Preparation Methods
Synthetic Routes and Reaction Conditions
The synthesis of Spirofylline involves several steps, starting with the preparation of the core structure, which is a spiro compound. The reaction conditions typically involve the use of organic solvents and catalysts to facilitate the formation of the spiro structure. The exact synthetic route can vary, but it generally includes steps such as cyclization and functional group modifications .
Industrial Production Methods
Industrial production of this compound may involve large-scale chemical reactors where the reaction conditions are carefully controlled to ensure high yield and purity. The process may include purification steps such as crystallization or chromatography to isolate the desired product .
Chemical Reactions Analysis
Types of Reactions
Spirofylline can undergo various chemical reactions, including:
Oxidation: This reaction involves the addition of oxygen or the removal of hydrogen, often using oxidizing agents such as hydrogen peroxide or potassium permanganate.
Reduction: This reaction involves the addition of hydrogen or the removal of oxygen, typically using reducing agents like sodium borohydride or lithium aluminum hydride.
Substitution: This reaction involves the replacement of one functional group with another, often using reagents such as halogens or nucleophiles.
Common Reagents and Conditions
Common reagents used in the reactions of this compound include oxidizing agents like hydrogen peroxide, reducing agents like sodium borohydride, and nucleophiles for substitution reactions. The reaction conditions can vary but often involve controlled temperatures and the use of solvents to facilitate the reactions .
Major Products Formed
The major products formed from the reactions of this compound depend on the type of reaction. For example, oxidation may produce oxygenated derivatives, while reduction may yield hydrogenated products. Substitution reactions can result in various substituted derivatives depending on the reagents used .
Scientific Research Applications
Spirofylline has a wide range of scientific research applications, including:
Chemistry: It is used as a model compound to study spiro compound synthesis and reactivity.
Biology: Researchers use this compound to investigate its effects on cellular respiration and its potential as a therapeutic agent.
Medicine: this compound is being studied for its potential to treat respiratory conditions such as asthma, bronchitis, and emphysema.
Industry: It is used in the development of new bronchodilator drugs and other pharmaceutical applications
Mechanism of Action
Spirofylline exerts its effects by targeting specific molecular pathways involved in bronchoconstriction. It works by relaxing the smooth muscles in the airways, leading to bronchodilation. The exact molecular targets and pathways involved include the inhibition of phosphodiesterase enzymes, which results in increased levels of cyclic adenosine monophosphate (cAMP) and subsequent relaxation of bronchial smooth muscles .
Comparison with Similar Compounds
Similar Compounds
Spirofylline is similar to other bronchodilator compounds such as theophylline and aminophylline. These compounds also target the phosphodiesterase enzymes and increase cAMP levels to achieve bronchodilation .
Uniqueness
What sets this compound apart from other similar compounds is its unique spiro structure, which may contribute to its specific pharmacokinetic and pharmacodynamic properties. This unique structure can affect its absorption, distribution, metabolism, and excretion, potentially offering advantages in terms of efficacy and safety .
Properties
IUPAC Name |
1,3-dimethyl-7-[2-oxo-2-[2-oxo-8-(2-phenylethyl)-1-oxa-3,8-diazaspiro[4.5]decan-3-yl]ethyl]purine-2,6-dione | |
---|---|---|
Source | PubChem | |
URL | https://pubchem.ncbi.nlm.nih.gov | |
Description | Data deposited in or computed by PubChem | |
InChI |
InChI=1S/C24H28N6O5/c1-26-20-19(21(32)27(2)22(26)33)29(16-25-20)14-18(31)30-15-24(35-23(30)34)9-12-28(13-10-24)11-8-17-6-4-3-5-7-17/h3-7,16H,8-15H2,1-2H3 | |
Source | PubChem | |
URL | https://pubchem.ncbi.nlm.nih.gov | |
Description | Data deposited in or computed by PubChem | |
InChI Key |
DSRGPEAMMDAUGF-UHFFFAOYSA-N | |
Source | PubChem | |
URL | https://pubchem.ncbi.nlm.nih.gov | |
Description | Data deposited in or computed by PubChem | |
Canonical SMILES |
CN1C2=C(C(=O)N(C1=O)C)N(C=N2)CC(=O)N3CC4(CCN(CC4)CCC5=CC=CC=C5)OC3=O | |
Source | PubChem | |
URL | https://pubchem.ncbi.nlm.nih.gov | |
Description | Data deposited in or computed by PubChem | |
Molecular Formula |
C24H28N6O5 | |
Source | PubChem | |
URL | https://pubchem.ncbi.nlm.nih.gov | |
Description | Data deposited in or computed by PubChem | |
DSSTOX Substance ID |
DTXSID60243512 | |
Record name | Spirofylline | |
Source | EPA DSSTox | |
URL | https://comptox.epa.gov/dashboard/DTXSID60243512 | |
Description | DSSTox provides a high quality public chemistry resource for supporting improved predictive toxicology. | |
Molecular Weight |
480.5 g/mol | |
Source | PubChem | |
URL | https://pubchem.ncbi.nlm.nih.gov | |
Description | Data deposited in or computed by PubChem | |
CAS No. |
98204-48-9 | |
Record name | Spirofylline [INN] | |
Source | ChemIDplus | |
URL | https://pubchem.ncbi.nlm.nih.gov/substance/?source=chemidplus&sourceid=0098204489 | |
Description | ChemIDplus is a free, web search system that provides access to the structure and nomenclature authority files used for the identification of chemical substances cited in National Library of Medicine (NLM) databases, including the TOXNET system. | |
Record name | Spirofylline | |
Source | EPA DSSTox | |
URL | https://comptox.epa.gov/dashboard/DTXSID60243512 | |
Description | DSSTox provides a high quality public chemistry resource for supporting improved predictive toxicology. | |
Record name | SPIROFYLLINE | |
Source | FDA Global Substance Registration System (GSRS) | |
URL | https://gsrs.ncats.nih.gov/ginas/app/beta/substances/000F949089 | |
Description | The FDA Global Substance Registration System (GSRS) enables the efficient and accurate exchange of information on what substances are in regulated products. Instead of relying on names, which vary across regulatory domains, countries, and regions, the GSRS knowledge base makes it possible for substances to be defined by standardized, scientific descriptions. | |
Explanation | Unless otherwise noted, the contents of the FDA website (www.fda.gov), both text and graphics, are not copyrighted. They are in the public domain and may be republished, reprinted and otherwise used freely by anyone without the need to obtain permission from FDA. Credit to the U.S. Food and Drug Administration as the source is appreciated but not required. | |
Retrosynthesis Analysis
AI-Powered Synthesis Planning: Our tool employs the Template_relevance Pistachio, Template_relevance Bkms_metabolic, Template_relevance Pistachio_ringbreaker, Template_relevance Reaxys, Template_relevance Reaxys_biocatalysis model, leveraging a vast database of chemical reactions to predict feasible synthetic routes.
One-Step Synthesis Focus: Specifically designed for one-step synthesis, it provides concise and direct routes for your target compounds, streamlining the synthesis process.
Accurate Predictions: Utilizing the extensive PISTACHIO, BKMS_METABOLIC, PISTACHIO_RINGBREAKER, REAXYS, REAXYS_BIOCATALYSIS database, our tool offers high-accuracy predictions, reflecting the latest in chemical research and data.
Strategy Settings
Precursor scoring | Relevance Heuristic |
---|---|
Min. plausibility | 0.01 |
Model | Template_relevance |
Template Set | Pistachio/Bkms_metabolic/Pistachio_ringbreaker/Reaxys/Reaxys_biocatalysis |
Top-N result to add to graph | 6 |
Feasible Synthetic Routes
Q1: The study highlights Spirofylline's drug-likeness based on Lipinski's rule of five. Could you explain the significance of this rule and how it applies to this compound in the context of COVID-19 treatment?
A1: Lipinski's rule of five is a set of guidelines used to assess the drug-likeness of a molecule, meaning its potential to be orally bioavailable. [, ] The rule states that for a compound to exhibit good oral absorption, it should ideally not violate more than one of the following criteria:
Disclaimer and Information on In-Vitro Research Products
Please be aware that all articles and product information presented on BenchChem are intended solely for informational purposes. The products available for purchase on BenchChem are specifically designed for in-vitro studies, which are conducted outside of living organisms. In-vitro studies, derived from the Latin term "in glass," involve experiments performed in controlled laboratory settings using cells or tissues. It is important to note that these products are not categorized as medicines or drugs, and they have not received approval from the FDA for the prevention, treatment, or cure of any medical condition, ailment, or disease. We must emphasize that any form of bodily introduction of these products into humans or animals is strictly prohibited by law. It is essential to adhere to these guidelines to ensure compliance with legal and ethical standards in research and experimentation.