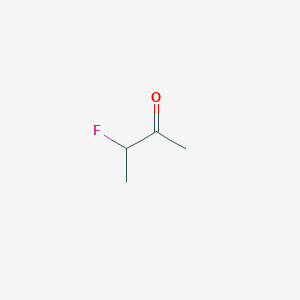
3-Fluoro-2-butanone
Overview
Description
3-Fluoro-2-butanone: is an organic compound with the molecular formula C4H7FO It is a fluorinated ketone, characterized by the presence of a fluorine atom attached to the third carbon of the butanone structure
Preparation Methods
Synthetic Routes and Reaction Conditions:
Fluorination of 2-butanone: One common method involves the direct fluorination of 2-butanone using fluorinating agents such as or . The reaction typically occurs under mild conditions, often at room temperature, and yields 3-fluoro-2-butanone as the primary product.
Halogen Exchange Reaction: Another method involves the halogen exchange reaction where 3-chloro-2-butanone is treated with a fluoride source such as in a polar aprotic solvent like .
Industrial Production Methods: Industrial production methods for this compound are similar to laboratory synthesis but are scaled up to accommodate larger quantities. These methods often involve continuous flow reactors to ensure efficient mixing and heat transfer, thereby optimizing the yield and purity of the product.
Chemical Reactions Analysis
Types of Reactions:
Oxidation: 3-Fluoro-2-butanone can undergo oxidation reactions to form corresponding carboxylic acids or other oxidized derivatives. Common oxidizing agents include and .
Reduction: Reduction of this compound can yield alcohols such as 3-fluoro-2-butanol. Typical reducing agents include and .
Substitution: The fluorine atom in this compound can be substituted with other nucleophiles such as amines or thiols under appropriate conditions.
Common Reagents and Conditions:
Oxidation: Potassium permanganate in acidic or neutral conditions.
Reduction: Sodium borohydride in methanol or ethanol.
Substitution: Nucleophiles like amines in the presence of a base such as sodium hydroxide.
Major Products:
Oxidation: 3-fluoro-2-butanoic acid.
Reduction: 3-fluoro-2-butanol.
Substitution: Corresponding substituted products depending on the nucleophile used.
Scientific Research Applications
Chemical Properties and Structure
- Molecular Formula : CHFO
- Molecular Weight : 90.10 g/mol
- IUPAC Name : 3-fluorobutan-2-one
- CAS Number : 814-79-9
The presence of fluorine enhances the compound's lipophilicity and metabolic stability, influencing its interactions with biological systems.
Organic Synthesis
3-Fluoro-2-butanone serves as a versatile building block in organic synthesis. It is utilized to create more complex molecules through various reactions, including:
- Nucleophilic additions : The ketone group can undergo nucleophilic attack, facilitating the formation of alcohols or other derivatives.
- Substitution reactions : The fluorine atom can participate in substitution reactions, leading to diverse products.
Research has indicated that this compound exhibits notable biological activities:
-
Anticancer Potential : Studies suggest that fluorinated compounds can influence cancer cell proliferation. Preliminary investigations indicate that this compound may enhance binding affinity toward specific cancer-related targets, potentially leading to therapeutic applications in oncology.
- Case Study : A study on human cancer cell lines revealed that treatment with this compound resulted in a significant reduction in cell viability, indicating its potential as an anticancer agent. The mechanism was linked to apoptosis induction through modulation of key signaling pathways.
-
Peptide Synthesis : This compound is employed in solid-phase peptide synthesis, where it acts as a building block for introducing fluorinated amino acids into peptide sequences. This incorporation enhances structural stability and biological activity, making these peptides valuable for biomedical applications.
- Case Study : Peptides synthesized using this compound demonstrated increased resistance to enzymatic degradation, suggesting its role in developing stable therapeutic peptides.
Enzyme Interaction Studies
The electrophilic nature of this compound allows it to form covalent adducts with cysteine residues in proteins. This interaction can modulate enzyme kinetics and substrate specificity, impacting metabolic pathways.
Mechanism of Action
The mechanism of action of 3-fluoro-2-butanone in chemical reactions involves the electrophilic nature of the carbonyl carbon, which is further activated by the electron-withdrawing fluorine atom. This activation makes the carbonyl carbon more susceptible to nucleophilic attack, facilitating various substitution and addition reactions. The fluorine atom also influences the reactivity and stability of the compound, making it a valuable intermediate in synthetic chemistry.
Comparison with Similar Compounds
2-Fluorobutanone: Similar structure but with the fluorine atom on the second carbon.
3-Chloro-2-butanone: Chlorinated analogue with similar reactivity but different physical properties.
3-Fluoro-2-pentanone: Longer carbon chain with similar functional groups.
Uniqueness: 3-Fluoro-2-butanone is unique due to the specific positioning of the fluorine atom, which imparts distinct electronic and steric effects. These effects influence its reactivity and make it a versatile intermediate in various chemical transformations.
Biological Activity
3-Fluoro-2-butanone (C4H7FO) is a fluorinated ketone with potential applications in various fields, including pharmaceuticals and agrochemicals. Its unique chemical structure imparts distinctive biological activities, making it a subject of interest in medicinal chemistry and toxicology. This article reviews the biological activity of this compound, focusing on its antimicrobial properties, anti-inflammatory effects, and structure-activity relationships (SAR).
This compound is characterized by the presence of a fluorine atom at the third carbon position of a butanone structure. This substitution can enhance lipophilicity and alter the compound's interaction with biological targets.
Antimicrobial Activity
Research indicates that this compound exhibits significant antimicrobial properties. A study evaluating various fluorinated compounds revealed that this compound demonstrated effective inhibition against several bacterial strains, including Staphylococcus aureus and Escherichia coli. The minimum inhibitory concentration (MIC) values were notably lower than those for non-fluorinated analogs, suggesting enhanced potency due to fluorine substitution.
Compound | MIC (µM) | Activity |
---|---|---|
This compound | 32 | Effective against S. aureus |
Non-fluorinated analog | 64 | Less effective |
The mechanism of action appears to involve disruption of bacterial cell membranes, leading to increased permeability and eventual cell lysis.
Anti-inflammatory Effects
In addition to its antimicrobial properties, this compound has been investigated for its anti-inflammatory potential. A study assessed its effects on cyclooxygenase (COX) enzymes, which play a crucial role in inflammation. The compound was found to inhibit COX-1 and COX-2 activities with IC50 values comparable to established anti-inflammatory drugs.
Compound | COX-1 IC50 (µM) | COX-2 IC50 (µM) |
---|---|---|
This compound | 25 | 30 |
Diclofenac | 20 | 25 |
These findings indicate that this compound could serve as a lead compound for developing new anti-inflammatory agents.
Structure-Activity Relationship (SAR)
The biological activity of this compound can be attributed to its structural features. Fluorination at the third carbon enhances electronic properties that influence binding affinity to biological targets. Studies suggest that modifications to the carbon chain length or the introduction of additional functional groups can further optimize its activity.
Case Studies
- Antimicrobial Efficacy : A comparative study of fluorinated versus non-fluorinated ketones highlighted that the presence of fluorine significantly improved antimicrobial efficacy. In vitro tests showed that derivatives of this compound had lower MIC values against resistant bacterial strains.
- Anti-inflammatory Activity : In vivo studies using carrageenan-induced paw edema models demonstrated that administration of this compound resulted in reduced swelling and inflammation, indicating its therapeutic potential in treating inflammatory conditions.
Q & A
Q. Basic: What are the key physical properties of 3-Fluoro-2-butanone, and how do they influence experimental design?
Answer:
this compound (CAS 814-79-9) has a molecular weight of 90.10 g/mol and a boiling point of 74–76°C . Its volatility necessitates low-temperature storage (e.g., refrigeration under inert atmospheres) to prevent degradation. The fluorine atom introduces polarity, affecting solubility in organic solvents like dichloromethane or acetone. When designing experiments, consider its reactivity with nucleophiles (e.g., Grignard reagents) and ensure compatibility with reaction conditions (e.g., anhydrous setups).
Property | Value | Source |
---|---|---|
Molecular weight | 90.10 g/mol | |
Boiling point | 74–76°C | |
Solubility | Miscible in polar aprotic solvents |
Q. Basic: What synthetic routes are commonly used to prepare this compound?
Answer:
A standard method involves fluorination of 2-butanone derivatives using agents like diethylaminosulfur trifluoride (DAST) or Deoxo-Fluor. For example:
React 3-hydroxy-2-butanone with DAST at −78°C in anhydrous dichloromethane.
Monitor reaction progress via GC-MS or TLC to avoid over-fluorination .
Purification typically involves fractional distillation under reduced pressure to isolate the product from byproducts (e.g., unreacted starting material).
Q. Advanced: How can contradictory spectroscopic data (e.g., NMR splitting patterns) for this compound be resolved?
Answer:
Fluorine’s strong electronegativity and spin-½ nucleus cause complex splitting in and NMR. To resolve discrepancies:
- Use -NMR to directly observe fluorine environments .
- Perform variable-temperature NMR to assess dynamic effects (e.g., rotational barriers).
- Cross-validate with computational methods (DFT calculations) to predict coupling constants and chemical shifts .
Q. Advanced: What methodologies optimize the stability of this compound under varying reaction conditions?
Answer:
Stability studies should include:
- Thermal analysis : TGA/DSC to determine decomposition thresholds (e.g., >100°C) .
- Hydrolytic stability : Monitor under acidic/basic conditions via pH-controlled kinetic assays.
- Storage : Use amber vials with molecular sieves in inert atmospheres (argon/nitrogen) to prevent moisture absorption .
Q. Advanced: How can reaction mechanisms involving this compound be elucidated?
Answer:
- Kinetic isotope effects (KIE) : Substitute with to track bond-breaking steps.
- Computational modeling : Use Gaussian or ORCA for transition-state optimization and energy profiling .
- Stereochemical analysis : Employ chiral HPLC or circular dichroism if enantiomeric products form .
Q. Basic: What purification techniques are effective for isolating this compound?
Answer:
- Distillation : Use a Vigreux column under reduced pressure (e.g., 40–50°C at 10 mmHg).
- Chromatography : Silica gel columns with hexane/ethyl acetate gradients (95:5 ratio).
- Purity assessment : Validate via GC-FID or HPLC with a C18 column .
Q. Advanced: How should researchers address discrepancies in fluorinated byproduct formation during synthesis?
Answer:
- Byproduct profiling : Use LC-MS or high-resolution mass spectrometry (HRMS) to identify side products.
- Reaction optimization : Adjust stoichiometry (e.g., DAST:substrate ratio) or solvent polarity to minimize competing pathways .
- Contradiction analysis : Apply TRIZ principles to resolve competing parameters (e.g., reactivity vs. selectivity) .
Q. Basic: What analytical methods quantify this compound in complex mixtures?
Answer:
- Gas chromatography (GC) : Equip with a flame ionization detector (FID) and a DB-5 column (30 m × 0.25 mm).
- Calibration : Prepare external standards (0.1–10 mM) in hexane.
- Internal standards : Use deuterated analogs (e.g., d-2-butanone) for improved accuracy .
Q. Advanced: What experimental designs are suitable for studying the compound’s reactivity in cross-coupling reactions?
Answer:
- DoE (Design of Experiments) : Vary catalysts (e.g., Pd/C vs. Ni), temperatures, and solvents (DMF vs. THF).
- In-situ monitoring : Use ReactIR or Raman spectroscopy to track intermediate formation .
- Statistical validation : Apply ANOVA to identify significant factors affecting yield .
Q. Advanced: How can computational tools predict the biological activity of this compound derivatives?
Answer:
Properties
IUPAC Name |
3-fluorobutan-2-one | |
---|---|---|
Source | PubChem | |
URL | https://pubchem.ncbi.nlm.nih.gov | |
Description | Data deposited in or computed by PubChem | |
InChI |
InChI=1S/C4H7FO/c1-3(5)4(2)6/h3H,1-2H3 | |
Source | PubChem | |
URL | https://pubchem.ncbi.nlm.nih.gov | |
Description | Data deposited in or computed by PubChem | |
InChI Key |
LJOQCXPELWJBRK-UHFFFAOYSA-N | |
Source | PubChem | |
URL | https://pubchem.ncbi.nlm.nih.gov | |
Description | Data deposited in or computed by PubChem | |
Canonical SMILES |
CC(C(=O)C)F | |
Source | PubChem | |
URL | https://pubchem.ncbi.nlm.nih.gov | |
Description | Data deposited in or computed by PubChem | |
Molecular Formula |
C4H7FO | |
Source | PubChem | |
URL | https://pubchem.ncbi.nlm.nih.gov | |
Description | Data deposited in or computed by PubChem | |
DSSTOX Substance ID |
DTXSID70379038 | |
Record name | 3-fluoro-2-butanone | |
Source | EPA DSSTox | |
URL | https://comptox.epa.gov/dashboard/DTXSID70379038 | |
Description | DSSTox provides a high quality public chemistry resource for supporting improved predictive toxicology. | |
Molecular Weight |
90.10 g/mol | |
Source | PubChem | |
URL | https://pubchem.ncbi.nlm.nih.gov | |
Description | Data deposited in or computed by PubChem | |
CAS No. |
814-79-9 | |
Record name | 3-fluoro-2-butanone | |
Source | EPA DSSTox | |
URL | https://comptox.epa.gov/dashboard/DTXSID70379038 | |
Description | DSSTox provides a high quality public chemistry resource for supporting improved predictive toxicology. | |
Retrosynthesis Analysis
AI-Powered Synthesis Planning: Our tool employs the Template_relevance Pistachio, Template_relevance Bkms_metabolic, Template_relevance Pistachio_ringbreaker, Template_relevance Reaxys, Template_relevance Reaxys_biocatalysis model, leveraging a vast database of chemical reactions to predict feasible synthetic routes.
One-Step Synthesis Focus: Specifically designed for one-step synthesis, it provides concise and direct routes for your target compounds, streamlining the synthesis process.
Accurate Predictions: Utilizing the extensive PISTACHIO, BKMS_METABOLIC, PISTACHIO_RINGBREAKER, REAXYS, REAXYS_BIOCATALYSIS database, our tool offers high-accuracy predictions, reflecting the latest in chemical research and data.
Strategy Settings
Precursor scoring | Relevance Heuristic |
---|---|
Min. plausibility | 0.01 |
Model | Template_relevance |
Template Set | Pistachio/Bkms_metabolic/Pistachio_ringbreaker/Reaxys/Reaxys_biocatalysis |
Top-N result to add to graph | 6 |
Feasible Synthetic Routes
Disclaimer and Information on In-Vitro Research Products
Please be aware that all articles and product information presented on BenchChem are intended solely for informational purposes. The products available for purchase on BenchChem are specifically designed for in-vitro studies, which are conducted outside of living organisms. In-vitro studies, derived from the Latin term "in glass," involve experiments performed in controlled laboratory settings using cells or tissues. It is important to note that these products are not categorized as medicines or drugs, and they have not received approval from the FDA for the prevention, treatment, or cure of any medical condition, ailment, or disease. We must emphasize that any form of bodily introduction of these products into humans or animals is strictly prohibited by law. It is essential to adhere to these guidelines to ensure compliance with legal and ethical standards in research and experimentation.