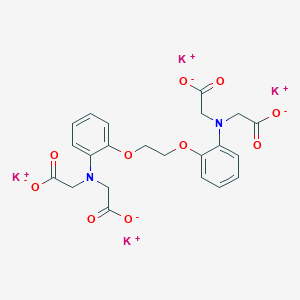
Potassium 2,2',2'',2'''-(((ethane-1,2-diylbis(oxy))bis(2,1-phenylene))bis(azanetriyl))tetraacetate
Overview
Description
Potassium 2,2',2'',2'''-(((ethane-1,2-diylbis(oxy))bis(2,1-phenylene))bis(azanetriyl))tetraacetate (CAS: 73630-08-7) is a potassium salt derivative of the calcium chelator BAPTA (2,2',2'',2'''-(Ethane-1,2-diylbis(oxy))bis(2,1-phenylene)bis(azanetriyl)tetraacetic acid). Its molecular formula is C₂₂H₂₀K₄N₂O₁₀ (MW: 628.79), featuring a rigid aromatic backbone with ethane-1,2-diylbis(oxy) linkers and four acetate groups for metal coordination . The potassium counterions enhance aqueous solubility, making it suitable for biological applications requiring precise calcium buffering .
Preparation Methods
Synthetic Routes and Reaction Conditions: BAPTA tetrapotassium is synthesized through a multi-step chemical process. The synthesis typically involves the reaction of 1,2-bis(o-aminophenoxy)ethane-N,N,N’,N’-tetraacetic acid (BAPTA) with potassium hydroxide to form the tetrapotassium salt. The reaction is carried out under controlled conditions to ensure high purity and yield .
Industrial Production Methods: Industrial production of BAPTA tetrapotassium involves large-scale synthesis using similar chemical routes as in laboratory settings. The process is optimized for efficiency, scalability, and cost-effectiveness. The final product is subjected to rigorous quality control measures to ensure its suitability for research applications .
Chemical Reactions Analysis
Calcium Chelation Reaction
BAPTA tetrapotassium salt exhibits high affinity for calcium ions (Ca²⁺), forming a 1:1 complex through coordination with its four carboxymethyl groups. The reaction is reversible and pH-dependent, with optimal binding occurring under neutral to slightly alkaline conditions .
Reaction Mechanism:
Key Properties:
-
Selectivity: BAPTA shows >10⁴-fold selectivity for Ca²⁺ over Mg²⁺, making it ideal for isolating calcium-dependent processes .
-
Kinetics: Rapid association/dissociation rates enable dynamic control of intracellular Ca²⁺ levels .
Applications:
-
Inhibition of calcium-dependent enzymes (e.g., calmodulin).
-
Real-time monitoring of calcium flux in neurons and muscle cells .
Interaction with Ester Derivatives
BAPTA tetrapotassium salt is generated via hydrolysis of its cell-permeable acetoxymethyl ester (BAPTA-AM). Intracellular esterases cleave the ester groups, releasing the active tetraanion .
Reaction Pathway:
Experimental Data:
Parameter | Value |
---|---|
Hydrolysis Rate | Complete within 30–60 min (37°C) |
Cellular Uptake | Enhanced by ester lipophilicity |
This reaction is critical for delivering BAPTA into cells without disrupting membrane integrity .
Fluorescence Quenching and Recovery
BAPTA’s fluorescence properties are modulated by Ca²⁺ binding. The unbound form exhibits weak fluorescence, which intensifies upon Ca²⁺ chelation .
Key Observations:
-
Emission Shift: → 20% increase in quantum yield upon Ca²⁺ binding.
Research Application:
Used in fluorescent probes (e.g., Fura-2 analogs) to quantify cytosolic Ca²⁺ concentrations in live cells .
Comparative Analysis with Other Chelators
BAPTA’s specificity distinguishes it from broader-spectrum chelators like EDTA and EGTA:
Chelator | Selectivity (Ca²⁺ vs. Mg²⁺) | (Ca²⁺) | Primary Use |
---|---|---|---|
BAPTA | >10⁴ | 0.1 nM | Intracellular Ca²⁺ control |
EGTA | ~200 | 150 nM | Extracellular Ca²⁺ buffering |
EDTA | Non-selective | 10 μM | Metal ion sequestration |
Role in Liposomal Drug Delivery
Recent studies demonstrate BAPTA’s utility in calcium-responsive liposomes. Incorporation of BAPTA derivatives into lipid bilayers enables triggered drug release upon exposure to elevated Ca²⁺ levels (e.g., in tumor microenvironments) .
Mechanism:
-
Ca²⁺ binding induces structural reorganization of liposomal membranes.
-
Release efficiency correlates with Ca²⁺ concentration (EC₅₀ ≈ 5 mM) .
Stability and Compatibility
Scientific Research Applications
Hazard Information
BAPTA tetrapotassium salt is classified with the following hazard statements:
- H302: Harmful if swallowed.
- H315: Causes skin irritation.
- H319: Causes serious eye irritation.
- H335: May cause respiratory irritation.
Precautionary measures include avoiding breathing dust and using protective eyewear and gloves.
Cell Biology
BAPTA is extensively utilized in cell biology to study intracellular calcium signaling. By chelating calcium ions, researchers can manipulate calcium levels within cells, allowing for the investigation of calcium-dependent processes such as muscle contraction, neurotransmitter release, and cell proliferation.
Case Study: Calcium Signaling in Neurons
In a study examining the role of calcium in neurotransmitter release, BAPTA was used to buffer intracellular calcium levels. The results demonstrated that reducing calcium availability significantly inhibited synaptic transmission in neurons, highlighting the compound's utility in understanding synaptic mechanisms .
Pharmacology
In pharmacological studies, BAPTA serves as a tool for assessing drug interactions that involve calcium channels. By inhibiting calcium influx, researchers can evaluate the efficacy of drugs targeting these channels.
Case Study: Drug Efficacy on Cardiac Cells
A research project investigated the effects of a new cardiac drug on calcium dynamics in heart cells. BAPTA was employed to control intracellular calcium levels, revealing that the drug improved contractility by enhancing calcium sensitivity in cardiac myocytes .
Toxicology
BAPTA has applications in toxicology for studying the effects of toxic substances on cellular calcium homeostasis. By chelating excess calcium, it can help elucidate mechanisms of toxicity related to calcium overload.
Case Study: Neurotoxicity Assessment
In neurotoxicology studies, BAPTA was used to assess the neurotoxic effects of heavy metals on neuronal cells. The findings indicated that heavy metal exposure led to increased intracellular calcium levels, which could be mitigated by BAPTA treatment, thereby reducing cell death .
Molecular Biology
In molecular biology, BAPTA is often used during experiments involving enzyme assays where calcium acts as a cofactor. By controlling calcium concentrations, researchers can optimize enzyme activity for various biochemical assays.
Case Study: Enzyme Activity Regulation
A study focused on calmodulin-dependent enzymes demonstrated that varying BAPTA concentrations allowed precise control over enzyme activity, leading to insights into enzyme kinetics and regulation mechanisms .
Comparative Data Table
Application Area | Purpose | Key Findings |
---|---|---|
Cell Biology | Manipulate intracellular Ca²⁺ | Inhibition of neurotransmitter release |
Pharmacology | Assess drug interactions | Improved contractility in cardiac cells |
Toxicology | Study toxic substance effects | Mitigation of heavy metal-induced neurotoxicity |
Molecular Biology | Optimize enzyme assays | Insights into enzyme kinetics |
Mechanism of Action
BAPTA tetrapotassium exerts its effects by binding to calcium ions with high affinity. This chelation process effectively reduces the concentration of free calcium ions in the solution, allowing researchers to control and manipulate calcium-dependent processes. The compound’s selectivity for calcium over magnesium is due to its specific chemical structure, which forms a stable complex with calcium ions .
Comparison with Similar Compounds
Structural and Functional Comparison with Similar Compounds
Key Chelating Agents and Their Properties
The table below compares the structural and functional attributes of the subject compound with EDTA, DTPA, BAPTA, and DOTA:
Structural Differences and Implications
- Rigidity vs. Flexibility : The subject compound and BAPTA contain aromatic phenylene groups and ethane-1,2-diylbis(oxy) linkers, conferring rigidity. This enhances selectivity for Ca²⁺ over Mg²⁺ compared to flexible ligands like EDTA .
- Coordination Geometry : EDTA and DTPA adopt flexible linear geometries, enabling binding to diverse metals. In contrast, the subject compound’s preorganized structure mimics natural Ca²⁺-binding proteins like calmodulin .
- Macrocyclic vs. Acyclic : DOTA’s macrocyclic structure provides superior thermodynamic stability for lanthanides, whereas the subject compound’s acyclic design prioritizes rapid Ca²⁺ binding/release kinetics .
Biological Activity
Potassium 2,2',2'',2'''-(((ethane-1,2-diylbis(oxy))bis(2,1-phenylene))bis(azanetriyl))tetraacetate, commonly referred to as Potassium BAPTA (CAS No. 73630-08-7), is a chelating agent with significant biological activity. This compound is primarily known for its ability to bind calcium ions (Ca²⁺) and is widely used in biochemistry and cell biology to manipulate intracellular calcium levels. This article discusses the biological activity of Potassium BAPTA, supported by data tables, case studies, and detailed research findings.
Molecular Structure
- Molecular Formula : C₂₂H₂₀K₄N₂O₁₀
- Molecular Weight : 628.80 g/mol
- InChIKey : MAWIDOKQOLSOFT-UHFFFAOYSA-J
Structural Representation
The compound features a complex structure with multiple functional groups that facilitate its chelating properties. The presence of ether and amine linkages enhances its stability and binding affinity for metal ions.
Calcium Chelation
Potassium BAPTA is renowned for its high affinity for calcium ions. It effectively sequesters Ca²⁺ ions, making it invaluable in various experimental settings:
- Intracellular Calcium Regulation : By buffering calcium levels, Potassium BAPTA can prevent calcium overload in cells, which is crucial during experiments involving calcium signaling pathways.
- Neurotransmitter Release : Studies have shown that Potassium BAPTA can inhibit neurotransmitter release in neurons by reducing intracellular calcium concentrations .
Case Studies
- Neuronal Studies : In a study examining the role of calcium in neurotransmitter release, Potassium BAPTA was used to demonstrate that lowering intracellular calcium levels significantly reduced synaptic transmission in hippocampal neurons .
- Cardiovascular Research : Research involving cardiac myocytes indicated that Potassium BAPTA could effectively reduce calcium-induced contractility, highlighting its potential therapeutic applications in managing cardiac hypertrophy .
- Cellular Signaling : A study focused on T-cell activation revealed that Potassium BAPTA inhibited calcium influx, thereby affecting T-cell proliferation and cytokine production .
The mechanism by which Potassium BAPTA exerts its biological effects primarily revolves around its ability to bind free calcium ions:
- Binding Affinity : The compound has a significantly higher affinity for Ca²⁺ compared to other divalent cations, which allows it to effectively compete with physiological calcium-binding proteins.
- Influence on Signaling Pathways : By modulating intracellular calcium levels, Potassium BAPTA influences various signaling pathways involved in cell growth, differentiation, and apoptosis.
Table 1: Comparative Binding Affinities of Calcium Chelators
Chelator | Binding Constant (K) | Specificity |
---|---|---|
Potassium BAPTA | High | Ca²⁺ > Mg²⁺ |
EDTA | Moderate | Ca²⁺ = Mg²⁺ |
EGTA | Moderate | Ca²⁺ >> Mg²⁺ |
Table 2: Summary of Biological Effects of Potassium BAPTA
Biological Effect | Observed Outcome |
---|---|
Inhibition of neurotransmitter release | Reduced synaptic transmission |
Modulation of cardiac contractility | Decreased contractile force |
Alteration of T-cell activation | Inhibited proliferation |
Q & A
Basic Research Questions
Q. What are the optimal synthetic routes for preparing Potassium 2,2',2'',2'''-(((ethane-1,2-diylbis(oxy))bis(2,1-phenylene))bis(azanetriyl))tetraacetate, and how can purity be maximized?
Methodological Answer: The synthesis involves multi-step condensation of ethane-1,2-diylbis(oxy)bis(2,1-phenylene) derivatives with glycine or its potassium salts under controlled pH (8–10) and temperature (60–80°C). Key steps include:
- Reaction Optimization : Use anhydrous solvents (e.g., DMF) to minimize hydrolysis of intermediates. Monitor reaction progress via TLC or HPLC .
- Purification : Recrystallize from ethanol/water mixtures to remove unreacted glycine or phenolic byproducts. Purity (>95%) can be verified via elemental analysis and NMR .
- Storage : Store under anhydrous conditions at room temperature to prevent deliquescence or decomposition .
Q. Which spectroscopic and crystallographic techniques are most effective for characterizing this compound?
Methodological Answer:
- FT-IR : Identify characteristic peaks for amide C=O (1650–1680 cm⁻¹), aromatic C-O (1250 cm⁻¹), and carboxylate groups (1400–1450 cm⁻¹) .
- NMR : Use ¹H/¹³C NMR in D₂O to resolve signals for the ethylene-dioxy bridge (δ 3.8–4.2 ppm) and aromatic protons (δ 6.5–7.5 ppm) .
- X-ray Crystallography : Analyze single crystals grown via slow evaporation (e.g., water/methanol). The structure reveals hydrogen bonding between carboxylate oxygens and water molecules, critical for stability .
Q. How does the compound’s stability vary under different pH and temperature conditions?
Methodological Answer:
- pH Stability : Conduct accelerated degradation studies in buffers (pH 2–12). The compound is stable at pH 6–8 but hydrolyzes in strong acid/base due to cleavage of the amide bonds .
- Thermal Stability : Perform TGA/DSC to identify decomposition onset (~220°C). Store below 25°C to avoid thermal degradation .
Advanced Research Questions
Q. How can this ligand be used to design coordination polymers, and what factors influence metal-binding selectivity?
Methodological Answer:
- Coordination Geometry : The tetracarboxylate groups enable octadentate binding to transition metals (e.g., Ni²⁺, Cu²⁺). Preferential binding to hard Lewis acids (e.g., Fe³⁺) is observed due to oxygen donor atoms .
- Synthesis of Polymers : React with metal salts (e.g., NiCl₂) in aqueous methanol (1:2 molar ratio). Characterize via PXRD and EXAFS to confirm 3D network formation .
- Tuning Selectivity : Adjust pH (5–7) and counterion (e.g., Cl⁻ vs. NO₃⁻) to modulate metal affinity .
Q. How can conflicting data on its chelation efficacy (e.g., vs. EDTA derivatives) be resolved?
Methodological Answer:
- Comparative Studies : Conduct potentiometric titrations to determine log K values for metal complexes (e.g., Ca²⁺, Mg²⁺). This ligand’s rigid aromatic backbone may reduce flexibility, lowering binding constants compared to EDTA .
- Mechanistic Analysis : Use DFT calculations to model steric effects and electron density distribution at binding sites. Experimental validation via UV-Vis titration (e.g., competition assays with EDTA) .
Q. What advanced spectroscopic methods can elucidate its role in metal-ion sensing or catalysis?
Methodological Answer:
- Fluorescence Quenching : Incorporate the ligand into lanthanide complexes (e.g., Eu³⁺) for ratiometric sensing. Monitor emission at 615 nm (λ_ex = 395 nm) upon analyte binding .
- EPR Spectroscopy : Study redox-active metal complexes (e.g., Cu²⁺) to probe electronic environments and spin states during catalytic cycles .
Q. How can degradation pathways be analyzed to improve its application in long-term studies?
Methodological Answer:
- LC-MS/MS : Identify hydrolysis products (e.g., phenolic fragments) under accelerated conditions (pH 1, 40°C). Quantify degradation kinetics using first-order models .
- Stabilization Strategies : Co-formulate with antioxidants (e.g., ascorbate) or encapsulate in silica nanoparticles to reduce oxidative/thermal degradation .
Properties
IUPAC Name |
tetrapotassium;2-[2-[2-[2-[bis(carboxylatomethyl)amino]phenoxy]ethoxy]-N-(carboxylatomethyl)anilino]acetate | |
---|---|---|
Source | PubChem | |
URL | https://pubchem.ncbi.nlm.nih.gov | |
Description | Data deposited in or computed by PubChem | |
InChI |
InChI=1S/C22H24N2O10.4K/c25-19(26)11-23(12-20(27)28)15-5-1-3-7-17(15)33-9-10-34-18-8-4-2-6-16(18)24(13-21(29)30)14-22(31)32;;;;/h1-8H,9-14H2,(H,25,26)(H,27,28)(H,29,30)(H,31,32);;;;/q;4*+1/p-4 | |
Source | PubChem | |
URL | https://pubchem.ncbi.nlm.nih.gov | |
Description | Data deposited in or computed by PubChem | |
InChI Key |
MAWIDOKQOLSOFT-UHFFFAOYSA-J | |
Source | PubChem | |
URL | https://pubchem.ncbi.nlm.nih.gov | |
Description | Data deposited in or computed by PubChem | |
Canonical SMILES |
C1=CC=C(C(=C1)N(CC(=O)[O-])CC(=O)[O-])OCCOC2=CC=CC=C2N(CC(=O)[O-])CC(=O)[O-].[K+].[K+].[K+].[K+] | |
Source | PubChem | |
URL | https://pubchem.ncbi.nlm.nih.gov | |
Description | Data deposited in or computed by PubChem | |
Molecular Formula |
C22H20K4N2O10 | |
Source | PubChem | |
URL | https://pubchem.ncbi.nlm.nih.gov | |
Description | Data deposited in or computed by PubChem | |
Related CAS |
85233-19-8 (Parent) | |
Record name | Glycine, N,N'-(1,2-ethanediylbis(oxy-2,1-phenylene))bis(N-(carboxymethyl)-, tetrapotassium salt | |
Source | ChemIDplus | |
URL | https://pubchem.ncbi.nlm.nih.gov/substance/?source=chemidplus&sourceid=0073630087 | |
Description | ChemIDplus is a free, web search system that provides access to the structure and nomenclature authority files used for the identification of chemical substances cited in National Library of Medicine (NLM) databases, including the TOXNET system. | |
DSSTOX Substance ID |
DTXSID20994432 | |
Record name | Tetrapotassium 2,2',2'',2'''-[ethane-1,2-diylbis(oxy-2,1-phenylenenitrilo)]tetraacetate | |
Source | EPA DSSTox | |
URL | https://comptox.epa.gov/dashboard/DTXSID20994432 | |
Description | DSSTox provides a high quality public chemistry resource for supporting improved predictive toxicology. | |
Molecular Weight |
628.8 g/mol | |
Source | PubChem | |
URL | https://pubchem.ncbi.nlm.nih.gov | |
Description | Data deposited in or computed by PubChem | |
CAS No. |
73630-08-7 | |
Record name | Glycine, N,N'-(1,2-ethanediylbis(oxy-2,1-phenylene))bis(N-(carboxymethyl)-, tetrapotassium salt | |
Source | ChemIDplus | |
URL | https://pubchem.ncbi.nlm.nih.gov/substance/?source=chemidplus&sourceid=0073630087 | |
Description | ChemIDplus is a free, web search system that provides access to the structure and nomenclature authority files used for the identification of chemical substances cited in National Library of Medicine (NLM) databases, including the TOXNET system. | |
Record name | Tetrapotassium 2,2',2'',2'''-[ethane-1,2-diylbis(oxy-2,1-phenylenenitrilo)]tetraacetate | |
Source | EPA DSSTox | |
URL | https://comptox.epa.gov/dashboard/DTXSID20994432 | |
Description | DSSTox provides a high quality public chemistry resource for supporting improved predictive toxicology. | |
Disclaimer and Information on In-Vitro Research Products
Please be aware that all articles and product information presented on BenchChem are intended solely for informational purposes. The products available for purchase on BenchChem are specifically designed for in-vitro studies, which are conducted outside of living organisms. In-vitro studies, derived from the Latin term "in glass," involve experiments performed in controlled laboratory settings using cells or tissues. It is important to note that these products are not categorized as medicines or drugs, and they have not received approval from the FDA for the prevention, treatment, or cure of any medical condition, ailment, or disease. We must emphasize that any form of bodily introduction of these products into humans or animals is strictly prohibited by law. It is essential to adhere to these guidelines to ensure compliance with legal and ethical standards in research and experimentation.