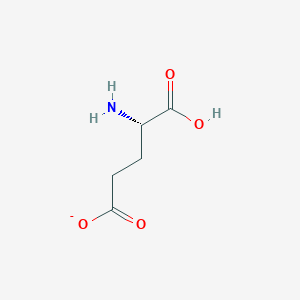
Sodium L-glutamate hydrate
Overview
Description
It is a white crystalline powder that is commonly used as a flavor enhancer in various cuisines, particularly in Asian cookingIt was first isolated and identified by Japanese biochemist Kikunae Ikeda in 1908 .
Mechanism of Action
Target of Action
The primary targets of Sodium (S)-2-amino-4-carboxybutanoate hydrate, also known as L-Glutamic acid monosodium salt hydrate, Sodium L-glutamate hydrate, or Monosodium Glutamate (MSG), are the glutamate receptors in the body . These receptors are found in various parts of the body, including the stomach, gut lining, and brain . They play a crucial role in transmitting signals in the nervous system and are involved in learning and memory .
Mode of Action
MSG acts as an excitatory neurotransmitter and an agonist at all subtypes of glutamate receptors, including metabotropic, kainate, NMDA, and AMPA receptors . It is absorbed through interaction with these receptors . Once in the gut, glutamate is either broken down to act as fuel or incorporated into other molecules .
Biochemical Pathways
MSG affects several biochemical pathways. It facilitates the binding of existing kokumi substances to kokumi receptors . In turn, these bound kokumi substances enhance the intensity of umami, sweet, salty, and fatty tastes, resulting in increased palatability accompanied by kokumi flavor, such as thickness, mouthfulness, and continuity .
Pharmacokinetics
The pharmacokinetics of MSG involve its absorption, distribution, metabolism, and excretion (ADME). After ingestion, MSG is absorbed in the gut through its interaction with glutamate receptors . It is then distributed throughout the body, where it can interact with various glutamate receptors. In the gut, MSG is either broken down to act as fuel or incorporated into other molecules .
Result of Action
The action of MSG results in a variety of molecular and cellular effects. It enhances the savory taste-active compounds when added in the proper concentration . It also increases the palatability of food, making it more enjoyable to eat . Furthermore, it has been suggested that high concentrations of MSG may increase the mitochondrial proton gradient, leading to the overproduction of mitochondrial superoxide .
Action Environment
Environmental factors can influence the action, efficacy, and stability of MSG. For instance, the concentration of MSG in a solution can affect its hydration and dynamics . Additionally, the presence of other substances, such as kokumi substances, can influence the action of MSG .
Biochemical Analysis
Biochemical Properties
Sodium (S)-2-amino-4-carboxybutanoate hydrate participates in various biochemical reactions. It interacts with numerous enzymes, proteins, and other biomolecules. As a derivative of glutamic acid, it is involved in protein synthesis and serves as a neurotransmitter in the central nervous system. It also plays a role in the citric acid cycle, contributing to energy production within cells .
Cellular Effects
The effects of Sodium (S)-2-amino-4-carboxybutanoate hydrate on cells and cellular processes are diverse. It influences cell function by impacting cell signaling pathways, gene expression, and cellular metabolism. For instance, as a neurotransmitter, it can activate signal transduction pathways leading to neuronal excitation .
Molecular Mechanism
At the molecular level, Sodium (S)-2-amino-4-carboxybutanoate hydrate exerts its effects through various mechanisms. It can bind to specific receptors on the cell surface, leading to the activation or inhibition of enzymes. Changes in gene expression can also occur as a result of its interaction with cellular components .
Temporal Effects in Laboratory Settings
In laboratory settings, the effects of Sodium (S)-2-amino-4-carboxybutanoate hydrate can change over time. It is stable under standard conditions, but its effects on cellular function may vary depending on the duration of exposure and the specific experimental conditions .
Dosage Effects in Animal Models
The effects of Sodium (S)-2-amino-4-carboxybutanoate hydrate in animal models can vary with different dosages. Lower doses may have minimal effects, while higher doses could lead to observable changes in physiological processes. Specific studies detailing these effects are currently limited .
Metabolic Pathways
Sodium (S)-2-amino-4-carboxybutanoate hydrate is involved in several metabolic pathways. As a derivative of glutamic acid, it participates in amino acid metabolism. It can also be involved in the citric acid cycle, contributing to the production of ATP, the primary energy currency of the cell .
Transport and Distribution
The transport and distribution of Sodium (S)-2-amino-4-carboxybutanoate hydrate within cells and tissues involve various transporters and binding proteins. It can be actively transported across cell membranes, and its distribution can be influenced by factors such as pH and ion concentration .
Subcellular Localization
The subcellular localization of Sodium (S)-2-amino-4-carboxybutanoate hydrate can influence its activity and function. It is primarily found in the cytoplasm, where it can participate in various metabolic processes. It can also be transported into organelles such as mitochondria, where it can contribute to energy production .
Preparation Methods
Monosodium glutamate can be produced through several methods:
Hydrolysis of Vegetable Proteins: This method involves the hydrolysis of vegetable proteins using hydrochloric acid to break peptide bonds and release glutamic acid, which is then neutralized with sodium hydroxide to form monosodium glutamate.
Chemical Synthesis: Monosodium glutamate can also be synthesized chemically using acrylonitrile.
Bacterial Fermentation: The most common industrial method for producing monosodium glutamate is bacterial fermentation. In this process, bacteria such as Corynebacterium species are cultured with ammonia and carbohydrates from sources like sugar beets, sugarcane, or molasses.
Chemical Reactions Analysis
Monosodium glutamate undergoes various chemical reactions:
Oxidation: Monosodium glutamate can be oxidized to form glutamic acid and other derivatives.
Dehydration: When heated to high temperatures, monosodium glutamate can undergo dehydration reactions to form pyroglutamate.
Substitution: Monosodium glutamate can participate in substitution reactions where the sodium ion is replaced by other cations.
Common reagents used in these reactions include hydrochloric acid, sodium hydroxide, and various oxidizing agents. The major products formed from these reactions include glutamic acid, pyroglutamate, and other glutamate derivatives.
Scientific Research Applications
Monosodium glutamate has a wide range of scientific research applications:
Chemistry: Monosodium glutamate is used as a reagent in various chemical reactions and studies involving amino acids and peptides.
Biology: In biological research, monosodium glutamate is used to study the effects of glutamate on cellular processes and neurotransmission.
Industry: Monosodium glutamate is widely used in the food industry as a flavor enhancer.
Comparison with Similar Compounds
Monosodium glutamate is unique among flavor enhancers due to its ability to impart the umami taste. Similar compounds include:
Disodium Inosinate: Often used in combination with monosodium glutamate to enhance umami flavor.
Disodium Guanylate: Another umami enhancer that is commonly used with monosodium glutamate.
Hydrolyzed Vegetable Protein: Contains glutamate and is used as a flavor enhancer in various foods.
Autolyzed Yeast Extract: Contains naturally occurring glutamate and is used to enhance flavor in processed foods.
Monosodium glutamate stands out due to its widespread use and effectiveness in enhancing the savory taste of foods.
Biological Activity
Sodium L-glutamate hydrate, commonly referred to as monosodium glutamate (MSG), is a sodium salt of the amino acid L-glutamic acid. It is widely used as a flavor enhancer in food and has various biological activities that have been the subject of extensive research. This article explores its biological activity, safety, and efficacy based on diverse sources.
- Molecular Formula : C₅H₈NNaO₄·xH₂O
- Molecular Weight : 169.1 g/mol
- Solubility : Highly soluble in water (417 g/L) .
Sodium L-glutamate functions primarily as an excitatory neurotransmitter in the central nervous system (CNS). It plays a critical role in synaptic transmission and plasticity, influencing learning and memory processes. The biological activity of glutamate is mediated through its interaction with various receptors, including:
- NMDA Receptors : Involved in synaptic plasticity and memory function.
- AMPA Receptors : Facilitate fast synaptic transmission.
- Metabotropic Glutamate Receptors (mGluRs) : Modulate neurotransmission and neuroprotection .
Biological Effects
- Neuroprotective Effects :
-
Toxicity and Safety :
- Acute toxicity studies reveal that sodium L-glutamate has low toxicity, with no adverse effects observed at doses up to 5,000 mg/kg body weight .
- Long-term studies indicate increased organ weights (kidney and spleen) at high doses; however, these changes were not associated with adverse histopathological findings .
- Potential Side Effects :
Table 1: Summary of Key Studies on this compound
Study | Findings | Methodology |
---|---|---|
Safety Assessment | No inhibition of microbial growth observed; low acute toxicity reported | Broth dilution method; animal studies |
Neuroprotective Study | Sodium L-glutamate enhances neuronal survival; mimics ischemic preconditioning | Isolated rat heart model |
Toxicological Evaluation | Increased organ weights observed but not adverse | OECD guideline studies on chronic toxicity |
Case Studies
-
Case Study on Neuroprotection :
In a study investigating the effects of sodium L-glutamate on rat cerebrocortical neurons, it was found that treatment with sodium L-glutamate improved cell viability under stress conditions. This suggests its potential role in neuroprotection during ischemic events . -
Epidemiological Observations :
Epidemiological studies have noted correlations between high MSG consumption and various health issues, including metabolic syndrome symptoms. However, these findings require further investigation to establish causation .
Properties
Key on ui mechanism of action |
L-Glutamate and GABA supposedly act as excitatory and inhibitory transmitters, respectively, in the central nervous system. Glutamate is also involved in the synthesis of proteins. /Glutamate/ |
---|---|
CAS No. |
6106-04-3 |
Molecular Formula |
C5H10NNaO5 |
Molecular Weight |
187.13 g/mol |
IUPAC Name |
sodium;(4S)-4-amino-5-hydroxy-5-oxopentanoate;hydrate |
InChI |
InChI=1S/C5H9NO4.Na.H2O/c6-3(5(9)10)1-2-4(7)8;;/h3H,1-2,6H2,(H,7,8)(H,9,10);;1H2/q;+1;/p-1/t3-;;/m0../s1 |
InChI Key |
GJBHGUUFMNITCI-QTNFYWBSSA-M |
impurities |
Monosodium glutamate is contaminated by sodium chloride, but as a food flavoring material this is generally of no consequence. Limits of impurities: Chlorides, 0.2%; Arsenic, 3 ppm (as As); Heavy metals, 20 ppm; Lead, 10 ppm |
SMILES |
C(CC(=O)O)C(C(=O)[O-])N.O.[Na+] |
Isomeric SMILES |
C(CC(=O)[O-])[C@@H](C(=O)O)N.O.[Na+] |
Canonical SMILES |
C(CC(=O)[O-])C(C(=O)O)N.O.[Na+] |
boiling_point |
225 °C (decomposes) |
Color/Form |
White free flowing crystals or crystalline powder Forms rhombic prisms when crystallized from wate |
density |
26.2 (saturated water solution at 20 °C) |
melting_point |
450 °F (Decomposes) (NTP, 1992) |
physical_description |
Monosodium glutamate appears as white or off-white crystalline powder with a slight peptone-like odor. pH (0.2% solution)7.0. (NTP, 1992) White, practically odourless crystals or crystalline powder White solid; [Merck Index] Fine colorless crystals; MSDSonline] |
solubility |
greater than or equal to 100 mg/mL at 68 °F (NTP, 1992) Freely soluble in water; practically insoluble in ethanol or ether SPARINGLY SOL IN ALCOHOL 73.9 G PER 100 ML WATER @ 25 °C; IT IS PRACTICALLY INSOL IN OIL OR ORGANIC SOLVENTS In water, 385,000 ppm at 25 °C |
Synonyms |
Aluminum L Glutamate Aluminum L-Glutamate D Glutamate D-Glutamate Glutamate Glutamate, Potassium Glutamic Acid Glutamic Acid, (D)-Isomer L Glutamate L Glutamic Acid L-Glutamate L-Glutamate, Aluminum L-Glutamic Acid Potassium Glutamate |
Origin of Product |
United States |
Retrosynthesis Analysis
AI-Powered Synthesis Planning: Our tool employs the Template_relevance Pistachio, Template_relevance Bkms_metabolic, Template_relevance Pistachio_ringbreaker, Template_relevance Reaxys, Template_relevance Reaxys_biocatalysis model, leveraging a vast database of chemical reactions to predict feasible synthetic routes.
One-Step Synthesis Focus: Specifically designed for one-step synthesis, it provides concise and direct routes for your target compounds, streamlining the synthesis process.
Accurate Predictions: Utilizing the extensive PISTACHIO, BKMS_METABOLIC, PISTACHIO_RINGBREAKER, REAXYS, REAXYS_BIOCATALYSIS database, our tool offers high-accuracy predictions, reflecting the latest in chemical research and data.
Strategy Settings
Precursor scoring | Relevance Heuristic |
---|---|
Min. plausibility | 0.01 |
Model | Template_relevance |
Template Set | Pistachio/Bkms_metabolic/Pistachio_ringbreaker/Reaxys/Reaxys_biocatalysis |
Top-N result to add to graph | 6 |
Feasible Synthetic Routes
Disclaimer and Information on In-Vitro Research Products
Please be aware that all articles and product information presented on BenchChem are intended solely for informational purposes. The products available for purchase on BenchChem are specifically designed for in-vitro studies, which are conducted outside of living organisms. In-vitro studies, derived from the Latin term "in glass," involve experiments performed in controlled laboratory settings using cells or tissues. It is important to note that these products are not categorized as medicines or drugs, and they have not received approval from the FDA for the prevention, treatment, or cure of any medical condition, ailment, or disease. We must emphasize that any form of bodily introduction of these products into humans or animals is strictly prohibited by law. It is essential to adhere to these guidelines to ensure compliance with legal and ethical standards in research and experimentation.