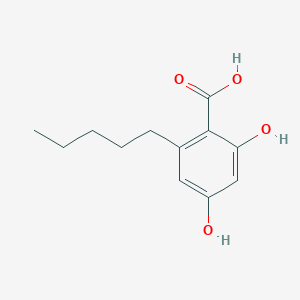
Olivetolic acid
Overview
Description
Olivetolic acid is a member of the class of benzoic acids. It is a salicylic acid in which the hydrogens ortho- and para- to the carboxy group are replaced by a pentyl and a hydroxy group, respectively . It has a role as a metabolite and is a monocarboxylic acid, a member of benzoic acids, a member of resorcinols, and a polyketide . It is functionally related to an olivetol .
Synthesis Analysis
The biosynthesis of Olivetolic acid has been studied in genetically engineered Yarrowia lipolytica . The study found that Pseudomonas sp LvaE encoding a short-chain acyl-CoA synthetase can efficiently convert hexanoic acid to hexanoyl-CoA . The co-expression of the acetyl-CoA carboxylase, the pyruvate dehydrogenase bypass, the NADPH-generating malic enzyme, as well as the activation of peroxisomal β-oxidation pathway and ATP export pathway are effective strategies to redirect carbon flux toward Olivetolic acid synthesis .Molecular Structure Analysis
The molecular formula of Olivetolic acid is C12H16O4 . Its molecular weight is 224.25 g/mol . The IUPAC name of Olivetolic acid is 2,4-dihydroxy-6-pentylbenzoic acid . The InChI and Canonical SMILES of Olivetolic acid are also available .Chemical Reactions Analysis
Olivetolic acid is a plant secondary metabolite sourced from PKS III catalysis . It is formed by an STCS-like enzyme through condensation of n-hexanoyl-CoA starter with three molecules of malonyl-CoA .Physical And Chemical Properties Analysis
The physical and chemical properties of Olivetolic acid can be found in the PubChem database . It is a member of benzoic acids, a member of resorcinols, and a polyketide .Scientific Research Applications
Cannabinoid Biosynthesis
OLA is a pivotal precursor in the biosynthesis of cannabinoids, which are compounds with significant neuroactive properties . The optimization of Olivetolic acid synthase (OLS), a key enzyme in this pathway, can enhance the production of cannabinoids like cannabidiol (CBD), which has various therapeutic applications.
Pharmaceutical Research
The biosynthetic pathways involving OLA are being explored for the production of pharmacologically active cannabinoids. These compounds are under clinical trials for their potential as anticancer agents, antiemetics, anticonvulsants, analgesics, and antidepressants .
Synthetic Biology
OLA serves as a building block in synthetic biology for the creation of complex molecules. Researchers have developed biosynthetic platforms that produce OLA and its analogues at high titers from microbes and in cell-free systems .
Enzyme Engineering
Studies on OLS have led to the identification of critical amino acid sites that significantly influence enzyme activity. This knowledge is crucial for enzyme engineering to improve the efficiency of OLA production .
Neuroscience
As a key intermediate in CBD synthesis, OLA is central to the study of cannabinoids’ effects on the nervous system. Cannabinoids interact with the human endocannabinoid system, influencing various neurological functions and conditions .
Metabolic Engineering
The metabolic pathways involving OLA are being engineered to increase the yield of desired cannabinoids. This includes modifying the expression of enzymes and introducing novel pathways in host organisms .
Chemical Synthesis
OLA and its derivatives are used in chemical synthesis as intermediates to create a wide range of cannabinoid-related compounds. This has implications for both research and commercial production of these substances.
Natural Product Chemistry
In natural product chemistry, OLA is studied for its role in the biosynthetic pathway of cannabinoids found in Cannabis sativa. Understanding this pathway is essential for the discovery and development of new natural products .
Safety And Hazards
When handling Olivetolic acid, it is recommended to avoid dust formation, avoid breathing mist, gas or vapours, avoid contacting with skin and eye, use personal protective equipment, wear chemical impermeable gloves, ensure adequate ventilation, remove all sources of ignition, evacuate personnel to safe areas, and keep people away from and upwind of spill/leak .
Future Directions
properties
IUPAC Name |
2,4-dihydroxy-6-pentylbenzoic acid | |
---|---|---|
Source | PubChem | |
URL | https://pubchem.ncbi.nlm.nih.gov | |
Description | Data deposited in or computed by PubChem | |
InChI |
InChI=1S/C12H16O4/c1-2-3-4-5-8-6-9(13)7-10(14)11(8)12(15)16/h6-7,13-14H,2-5H2,1H3,(H,15,16) | |
Source | PubChem | |
URL | https://pubchem.ncbi.nlm.nih.gov | |
Description | Data deposited in or computed by PubChem | |
InChI Key |
SXFKFRRXJUJGSS-UHFFFAOYSA-N | |
Source | PubChem | |
URL | https://pubchem.ncbi.nlm.nih.gov | |
Description | Data deposited in or computed by PubChem | |
Canonical SMILES |
CCCCCC1=C(C(=CC(=C1)O)O)C(=O)O | |
Source | PubChem | |
URL | https://pubchem.ncbi.nlm.nih.gov | |
Description | Data deposited in or computed by PubChem | |
Molecular Formula |
C12H16O4 | |
Source | PubChem | |
URL | https://pubchem.ncbi.nlm.nih.gov | |
Description | Data deposited in or computed by PubChem | |
DSSTOX Substance ID |
DTXSID20197688 | |
Record name | Olivetolic acid | |
Source | EPA DSSTox | |
URL | https://comptox.epa.gov/dashboard/DTXSID20197688 | |
Description | DSSTox provides a high quality public chemistry resource for supporting improved predictive toxicology. | |
Molecular Weight |
224.25 g/mol | |
Source | PubChem | |
URL | https://pubchem.ncbi.nlm.nih.gov | |
Description | Data deposited in or computed by PubChem | |
Product Name |
Olivetolic acid | |
CAS RN |
491-72-5 | |
Record name | Olivetolic acid | |
Source | CAS Common Chemistry | |
URL | https://commonchemistry.cas.org/detail?cas_rn=491-72-5 | |
Description | CAS Common Chemistry is an open community resource for accessing chemical information. Nearly 500,000 chemical substances from CAS REGISTRY cover areas of community interest, including common and frequently regulated chemicals, and those relevant to high school and undergraduate chemistry classes. This chemical information, curated by our expert scientists, is provided in alignment with our mission as a division of the American Chemical Society. | |
Explanation | The data from CAS Common Chemistry is provided under a CC-BY-NC 4.0 license, unless otherwise stated. | |
Record name | Olivetolic acid | |
Source | ChemIDplus | |
URL | https://pubchem.ncbi.nlm.nih.gov/substance/?source=chemidplus&sourceid=0000491725 | |
Description | ChemIDplus is a free, web search system that provides access to the structure and nomenclature authority files used for the identification of chemical substances cited in National Library of Medicine (NLM) databases, including the TOXNET system. | |
Record name | Olivetolic acid | |
Source | EPA DSSTox | |
URL | https://comptox.epa.gov/dashboard/DTXSID20197688 | |
Description | DSSTox provides a high quality public chemistry resource for supporting improved predictive toxicology. | |
Record name | OLIVETOLIC ACID | |
Source | FDA Global Substance Registration System (GSRS) | |
URL | https://gsrs.ncats.nih.gov/ginas/app/beta/substances/3Y8J575L93 | |
Description | The FDA Global Substance Registration System (GSRS) enables the efficient and accurate exchange of information on what substances are in regulated products. Instead of relying on names, which vary across regulatory domains, countries, and regions, the GSRS knowledge base makes it possible for substances to be defined by standardized, scientific descriptions. | |
Explanation | Unless otherwise noted, the contents of the FDA website (www.fda.gov), both text and graphics, are not copyrighted. They are in the public domain and may be republished, reprinted and otherwise used freely by anyone without the need to obtain permission from FDA. Credit to the U.S. Food and Drug Administration as the source is appreciated but not required. | |
Retrosynthesis Analysis
AI-Powered Synthesis Planning: Our tool employs the Template_relevance Pistachio, Template_relevance Bkms_metabolic, Template_relevance Pistachio_ringbreaker, Template_relevance Reaxys, Template_relevance Reaxys_biocatalysis model, leveraging a vast database of chemical reactions to predict feasible synthetic routes.
One-Step Synthesis Focus: Specifically designed for one-step synthesis, it provides concise and direct routes for your target compounds, streamlining the synthesis process.
Accurate Predictions: Utilizing the extensive PISTACHIO, BKMS_METABOLIC, PISTACHIO_RINGBREAKER, REAXYS, REAXYS_BIOCATALYSIS database, our tool offers high-accuracy predictions, reflecting the latest in chemical research and data.
Strategy Settings
Precursor scoring | Relevance Heuristic |
---|---|
Min. plausibility | 0.01 |
Model | Template_relevance |
Template Set | Pistachio/Bkms_metabolic/Pistachio_ringbreaker/Reaxys/Reaxys_biocatalysis |
Top-N result to add to graph | 6 |
Feasible Synthetic Routes
Q & A
Q1: What is the role of olivetolic acid in cannabinoid biosynthesis?
A1: Olivetolic acid serves as the precursor for the aromatic moiety of cannabinoids. It combines with geranyl diphosphate through the action of cannabigerolic acid synthase (CBGAS) to form cannabigerolic acid (CBGA), the central precursor to various cannabinoids like Δ9-tetrahydrocannabinolic acid (THCA) and cannabidiolic acid (CBDA) [, , , , , , , ].
Q2: How is olivetolic acid synthesized in Cannabis sativa?
A2: OA biosynthesis involves a two-step enzymatic process. First, tetraketide synthase (TKS), a type III polyketide synthase, uses malonyl-CoA and hexanoyl-CoA to produce a linear tetraketide intermediate [, , , ]. This intermediate is then cyclized by olivetolic acid cyclase (OAC) to form OA [, , , , , , , ].
Q3: Can olivetolic acid be produced in organisms other than Cannabis sativa?
A3: Yes, researchers have successfully engineered microorganisms like Escherichia coli [], Saccharomyces cerevisiae [, ], Yarrowia lipolytica [], Phaeodactylum tricornutum [], Aspergillus nidulans [], and even the amoeba Dictyostelium discoideum [] to produce OA. This highlights the potential for alternative, sustainable production platforms for this valuable compound.
Q4: What are the challenges and strategies for improving olivetolic acid production in heterologous hosts?
A4: Challenges include low production titers, shunt product formation, and the availability of precursors like hexanoyl-CoA and malonyl-CoA [, , ]. Strategies to overcome these limitations involve optimizing gene expression, engineering enzyme activity, and modifying metabolic pathways to enhance precursor supply [, , , ]. For instance, the fusion of enzymes involved in hexanoyl-CoA production with OA biosynthetic enzymes significantly increased OA production in E. coli [].
Q5: What is the significance of identifying genes involved in olivetolic acid biosynthesis?
A5: Identifying and characterizing genes like OLS, OAC, and CBGAS provides a foundation for understanding the genetic basis of cannabinoid production. This knowledge can be applied to develop molecular markers for breeding programs, optimize cultivation practices, and engineer cannabis plants with desired cannabinoid profiles [, , ].
Q6: What is the molecular formula and weight of olivetolic acid?
A6: The molecular formula of olivetolic acid is C12H16O4, and its molecular weight is 224.25 g/mol [].
Q7: What are the structural features of olivetolic acid?
A7: Olivetolic acid is an alkylresorcinolic acid characterized by a benzene ring with a pentyl chain at the C-1 position and hydroxyl groups at the C-2 and C-4 positions [, , ]. This unique structure contributes to its reactivity and biological activity.
Q8: Have any structural analogs of olivetolic acid been synthesized?
A8: Yes, researchers have successfully engineered enzymes like olivetolic acid cyclase (OAC) and tetraketide synthase (TKS) to generate OA analogs with varying alkyl chain lengths []. These analogs offer a platform for exploring the structure-activity relationship of OA and developing potentially more potent or selective cannabinoids.
Q9: What is the significance of the pentyl side chain in olivetolic acid?
A9: The length and structure of the alkyl chain at the C-3 position of cannabinoids, derived from the pentyl side chain of OA, significantly influence their pharmacological activities [, ]. Modifying this side chain through enzyme engineering could lead to novel cannabinoid analogs with altered potency and therapeutic potential.
Q10: Does olivetolic acid itself possess any biological activity?
A10: Research suggests that OA and its alkyl chain derivatives display antibacterial activity against Bacillus subtilis and Staphylococcus aureus [, ]. This finding highlights the potential of exploring OA and its analogs for novel antimicrobial agents.
Q11: What is the potential of olivetolic acid for developing novel cannabinoids?
A11: OA serves as a crucial building block for cannabinoid synthesis. By engineering the enzymes involved in OA biosynthesis and its subsequent conversion to cannabinoids, researchers can generate novel cannabinoid analogs with tailored pharmacological properties for therapeutic applications [, , ].
Q12: Has olivetolic acid been investigated for other applications besides cannabinoid synthesis?
A12: Yes, a recent study demonstrated the anticonvulsant effect of OA in a mouse model of Dravet syndrome, suggesting its potential therapeutic application beyond cannabinoids [].
Q13: Are there any natural sources of olivetolic acid other than Cannabis sativa?
A13: Olivetolic acid and its derivatives have been identified in other plant species, including Cetrelia sanguinea [] and Cladonia portentosa []. This discovery opens up possibilities for exploring alternative natural sources for this valuable compound.
Disclaimer and Information on In-Vitro Research Products
Please be aware that all articles and product information presented on BenchChem are intended solely for informational purposes. The products available for purchase on BenchChem are specifically designed for in-vitro studies, which are conducted outside of living organisms. In-vitro studies, derived from the Latin term "in glass," involve experiments performed in controlled laboratory settings using cells or tissues. It is important to note that these products are not categorized as medicines or drugs, and they have not received approval from the FDA for the prevention, treatment, or cure of any medical condition, ailment, or disease. We must emphasize that any form of bodily introduction of these products into humans or animals is strictly prohibited by law. It is essential to adhere to these guidelines to ensure compliance with legal and ethical standards in research and experimentation.