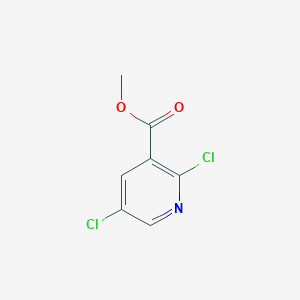
Methyl 2,5-dichloronicotinate
Overview
Description
Methyl 2,5-dichloronicotinate, also known as 2,5-dichloronicotinic acid methyl ester, is a halogenated heterocyclic compound. It is a derivative of nicotinic acid and has the molecular formula C7H5Cl2NO2. This compound is primarily used as an intermediate in organic synthesis and has applications in various fields, including pharmaceuticals and agrochemicals .
Preparation Methods
Synthetic Routes and Reaction Conditions
Methyl 2,5-dichloronicotinate can be synthesized through several methods. One common synthetic route involves the esterification of 2,5-dichloronicotinic acid with methanol in the presence of a catalyst such as sulfuric acid. The reaction typically occurs under reflux conditions, and the product is purified through recrystallization .
Industrial Production Methods
In industrial settings, the production of this compound often involves the use of continuous flow reactors to optimize yield and efficiency. The reaction conditions are carefully controlled to ensure high purity and consistent quality of the final product .
Chemical Reactions Analysis
Types of Reactions
Methyl 2,5-dichloronicotinate undergoes various chemical reactions, including:
Substitution Reactions: The chlorine atoms in the compound can be substituted with other nucleophiles, such as amines or thiols, under appropriate conditions.
Reduction Reactions: The compound can be reduced to form corresponding amines or alcohols using reducing agents like lithium aluminum hydride.
Oxidation Reactions: Oxidation of this compound can lead to the formation of carboxylic acids or other oxidized derivatives.
Common Reagents and Conditions
Substitution: Nucleophiles like amines or thiols in the presence of a base such as sodium hydroxide.
Reduction: Reducing agents like lithium aluminum hydride or sodium borohydride.
Oxidation: Oxidizing agents like potassium permanganate or chromium trioxide.
Major Products Formed
Substitution: Formation of substituted nicotinates.
Reduction: Formation of amines or alcohols.
Oxidation: Formation of carboxylic acids or other oxidized derivatives.
Scientific Research Applications
Methyl 2,5-dichloronicotinate has several applications in scientific research:
Chemistry: Used as an intermediate in the synthesis of various organic compounds.
Biology: Investigated for its potential biological activities, including antimicrobial and anticancer properties.
Medicine: Explored as a precursor for the development of pharmaceutical agents.
Industry: Utilized in the production of agrochemicals and other industrial chemicals
Mechanism of Action
The mechanism of action of methyl 2,5-dichloronicotinate is not fully understood. it is believed to interact with specific molecular targets and pathways, leading to its observed biological effects. The compound may exert its effects through the modulation of enzyme activity or interaction with cellular receptors .
Comparison with Similar Compounds
Similar Compounds
Methyl nicotinate: A methyl ester of nicotinic acid used as a rubefacient in topical preparations.
2,5-Dichloronicotinic acid: The parent acid of methyl 2,5-dichloronicotinate.
Methyl 3,5-dichloronicotinate: A similar compound with chlorine atoms at different positions on the nicotinic acid ring
Uniqueness
This compound is unique due to its specific substitution pattern on the nicotinic acid ring, which imparts distinct chemical and biological properties. This uniqueness makes it valuable in various synthetic and research applications .
Properties
IUPAC Name |
methyl 2,5-dichloropyridine-3-carboxylate | |
---|---|---|
Source | PubChem | |
URL | https://pubchem.ncbi.nlm.nih.gov | |
Description | Data deposited in or computed by PubChem | |
InChI |
InChI=1S/C7H5Cl2NO2/c1-12-7(11)5-2-4(8)3-10-6(5)9/h2-3H,1H3 | |
Source | PubChem | |
URL | https://pubchem.ncbi.nlm.nih.gov | |
Description | Data deposited in or computed by PubChem | |
InChI Key |
CNPFXYWTMBTSSI-UHFFFAOYSA-N | |
Source | PubChem | |
URL | https://pubchem.ncbi.nlm.nih.gov | |
Description | Data deposited in or computed by PubChem | |
Canonical SMILES |
COC(=O)C1=C(N=CC(=C1)Cl)Cl | |
Source | PubChem | |
URL | https://pubchem.ncbi.nlm.nih.gov | |
Description | Data deposited in or computed by PubChem | |
Molecular Formula |
C7H5Cl2NO2 | |
Source | PubChem | |
URL | https://pubchem.ncbi.nlm.nih.gov | |
Description | Data deposited in or computed by PubChem | |
DSSTOX Substance ID |
DTXSID40377400 | |
Record name | methyl 2,5-dichloronicotinate | |
Source | EPA DSSTox | |
URL | https://comptox.epa.gov/dashboard/DTXSID40377400 | |
Description | DSSTox provides a high quality public chemistry resource for supporting improved predictive toxicology. | |
Molecular Weight |
206.02 g/mol | |
Source | PubChem | |
URL | https://pubchem.ncbi.nlm.nih.gov | |
Description | Data deposited in or computed by PubChem | |
CAS No. |
67754-03-4 | |
Record name | methyl 2,5-dichloronicotinate | |
Source | EPA DSSTox | |
URL | https://comptox.epa.gov/dashboard/DTXSID40377400 | |
Description | DSSTox provides a high quality public chemistry resource for supporting improved predictive toxicology. | |
Record name | Methyl 2,5-Dichloronicotinate | |
Source | European Chemicals Agency (ECHA) | |
URL | https://echa.europa.eu/information-on-chemicals | |
Description | The European Chemicals Agency (ECHA) is an agency of the European Union which is the driving force among regulatory authorities in implementing the EU's groundbreaking chemicals legislation for the benefit of human health and the environment as well as for innovation and competitiveness. | |
Explanation | Use of the information, documents and data from the ECHA website is subject to the terms and conditions of this Legal Notice, and subject to other binding limitations provided for under applicable law, the information, documents and data made available on the ECHA website may be reproduced, distributed and/or used, totally or in part, for non-commercial purposes provided that ECHA is acknowledged as the source: "Source: European Chemicals Agency, http://echa.europa.eu/". Such acknowledgement must be included in each copy of the material. ECHA permits and encourages organisations and individuals to create links to the ECHA website under the following cumulative conditions: Links can only be made to webpages that provide a link to the Legal Notice page. | |
Synthesis routes and methods I
Procedure details
Synthesis routes and methods II
Procedure details
Retrosynthesis Analysis
AI-Powered Synthesis Planning: Our tool employs the Template_relevance Pistachio, Template_relevance Bkms_metabolic, Template_relevance Pistachio_ringbreaker, Template_relevance Reaxys, Template_relevance Reaxys_biocatalysis model, leveraging a vast database of chemical reactions to predict feasible synthetic routes.
One-Step Synthesis Focus: Specifically designed for one-step synthesis, it provides concise and direct routes for your target compounds, streamlining the synthesis process.
Accurate Predictions: Utilizing the extensive PISTACHIO, BKMS_METABOLIC, PISTACHIO_RINGBREAKER, REAXYS, REAXYS_BIOCATALYSIS database, our tool offers high-accuracy predictions, reflecting the latest in chemical research and data.
Strategy Settings
Precursor scoring | Relevance Heuristic |
---|---|
Min. plausibility | 0.01 |
Model | Template_relevance |
Template Set | Pistachio/Bkms_metabolic/Pistachio_ringbreaker/Reaxys/Reaxys_biocatalysis |
Top-N result to add to graph | 6 |
Feasible Synthetic Routes
Disclaimer and Information on In-Vitro Research Products
Please be aware that all articles and product information presented on BenchChem are intended solely for informational purposes. The products available for purchase on BenchChem are specifically designed for in-vitro studies, which are conducted outside of living organisms. In-vitro studies, derived from the Latin term "in glass," involve experiments performed in controlled laboratory settings using cells or tissues. It is important to note that these products are not categorized as medicines or drugs, and they have not received approval from the FDA for the prevention, treatment, or cure of any medical condition, ailment, or disease. We must emphasize that any form of bodily introduction of these products into humans or animals is strictly prohibited by law. It is essential to adhere to these guidelines to ensure compliance with legal and ethical standards in research and experimentation.