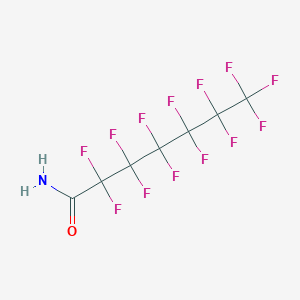
Tridecafluoroheptanamide
Overview
Description
Tridecafluoroheptanamide (2,2,3,3,4,4,5,5,6,6,7,7,7-tridecafluoroheptanamide; CAS 2358-22-7) is a perfluorinated amide with the molecular formula C₇H₂F₁₃NO and a molecular weight of 363.08 g/mol . It is characterized by a heptane backbone fully substituted with fluorine atoms except for the terminal amide group. Key physical properties include:
Preparation Methods
Synthetic Routes and Reaction Conditions
Tridecafluoroheptanamide can be synthesized through several methods. One common approach involves the reaction of perfluoroheptanoic acid with ammonia or an amine under controlled conditions. The reaction typically requires a catalyst and is carried out at elevated temperatures to facilitate the formation of the amide bond.
Industrial Production Methods
In industrial settings, the production of perfluoroheptanamide often involves the use of specialized equipment to handle the highly reactive and corrosive nature of perfluorinated compounds. The process may include steps such as distillation, purification, and quality control to ensure the final product meets the required specifications.
Chemical Reactions Analysis
Types of Reactions
Tridecafluoroheptanamide undergoes various chemical reactions, including:
Oxidation: This reaction can lead to the formation of perfluorinated carboxylic acids.
Reduction: Reduction reactions can convert the amide group to an amine.
Substitution: The fluorine atoms in the perfluorinated chain can be substituted with other functional groups under specific conditions.
Common Reagents and Conditions
Oxidation: Strong oxidizing agents such as potassium permanganate or ozone are commonly used.
Reduction: Reducing agents like lithium aluminum hydride or hydrogen gas in the presence of a catalyst can be employed.
Substitution: Nucleophilic reagents such as alkoxides or thiolates can be used for substitution reactions.
Major Products Formed
Oxidation: Perfluoroheptanoic acid.
Reduction: Perfluoroheptylamine.
Substitution: Various substituted perfluoroheptanamides depending on the nucleophile used.
Scientific Research Applications
Materials Science
Tridecafluoroheptanamide is utilized in the development of advanced materials, particularly in coatings and surface treatments. Its fluorinated structure imparts hydrophobic and oleophobic characteristics to surfaces, making it valuable for:
- Anti-fogging coatings: Used in optical devices and safety equipment.
- Self-cleaning surfaces: Applied in architectural materials to reduce maintenance needs.
Pharmaceuticals
The compound is being investigated for its potential use in drug formulation and delivery systems. Its unique properties can enhance the solubility and bioavailability of pharmaceutical compounds. Specific applications include:
- Drug solubilization: Fluorinated compounds often exhibit improved solubility profiles, which can be beneficial for poorly soluble drugs.
- Targeted delivery systems: The chemical structure can be modified to facilitate targeted drug delivery, enhancing therapeutic efficacy while minimizing side effects.
Environmental Studies
This compound is also explored for its environmental applications, particularly in the context of pollution control and remediation:
- Fluorinated surfactants: These compounds are effective in reducing surface tension and can be used to enhance the recovery of oil spills or other contaminants.
- Environmental monitoring: Its stability allows for its use as a tracer in studies assessing the movement of pollutants through various ecosystems.
Case Study 1: Coatings Technology
A study demonstrated that this compound-based coatings significantly improved the durability and performance of surfaces exposed to harsh environmental conditions. The coatings exhibited exceptional water repellency and resistance to staining, making them ideal for outdoor applications.
Case Study 2: Pharmaceutical Formulations
Research involving this compound as an excipient showed enhanced dissolution rates for several active pharmaceutical ingredients (APIs). The study concluded that incorporating this compound into formulations could lead to more effective drug delivery systems, particularly for oral medications.
Mechanism of Action
The mechanism of action of perfluoroheptanamide is primarily related to its interaction with biological membranes and proteins. The perfluorinated chain imparts hydrophobicity, allowing the compound to interact with lipid bilayers and alter membrane properties. Additionally, the amide group can form hydrogen bonds with proteins, potentially affecting their structure and function. These interactions can influence various molecular pathways and cellular processes.
Comparison with Similar Compounds
Comparison with Similar Compounds
Tridecafluoroheptanamide belongs to the broader class of per- and polyfluoroalkyl substances (PFAS), which share carbon-fluorine bonds but differ in functional groups and chain lengths. Below is a structured comparison with structurally or functionally related compounds:
Functional Group Variations
Sulfonamide PFAS (e.g., perfluorooctanesulfonamide, PFOSA):
- Key difference : Sulfonamide (-SO₂NH₂) vs. amide (-CONH₂) groups.
- Sulfonamides exhibit stronger acidity (due to -SO₂ group) and higher persistence in environmental matrices compared to amides. highlights sulfonamide PFAS developmental toxicity but lacks direct comparison with amide analogs .
- Implication : this compound’s amide group may reduce environmental mobility compared to sulfonamides but increase reactivity in hydrolysis.
- Phosphazene Derivatives (e.g., dispirophosphazenes from ): Key difference: Inorganic phosphorus-nitrogen backbone vs. organic fluorinated carbon chain. Phosphazenes (e.g., compounds 6a/6b in ) are thermally stable and used in flame retardants or elastomers. This compound’s fluorocarbon chain likely enhances hydrophobicity but reduces thermal stability compared to phosphazenes .
Chain-Length and Fluorination Patterns
- This compound’s C7 chain and 13 fluorines balance lipophilicity (LogP 3.91) and moderate volatility (vapor pressure: 1.26 mmHg at 25°C) .
- Longer-Chain PFAS (e.g., perfluorooctanoic acid, PFOA): PFOA (C8) has higher bioaccumulation and toxicity but lower thermal stability (decomposes above 190°C). This compound’s amide group may introduce hydrogen-bonding interactions absent in PFOA, altering environmental adsorption.
Physicochemical Properties
Toxicological and Environmental Profiles
- notes that sulfonamide PFAS induce transcriptional changes in developmental toxicity models, but comparative data for amide-based PFAS like this compound are absent .
- The amide group in this compound may mitigate metabolic persistence compared to sulfonamides, though its high fluorine content raises concerns about bioaccumulation .
Biological Activity
Tridecafluoroheptanamide (TDHA) is a perfluorinated compound that has garnered attention in various scientific fields, particularly due to its unique chemical properties and potential biological activities. This article explores the biological activity of TDHA, including its interactions with biological systems, potential applications, and relevant case studies.
Chemical Structure and Properties
This compound is characterized by a long perfluorinated chain, which significantly influences its solubility and interaction with biological membranes. The chemical formula is , indicating a complex structure that contributes to its stability and persistence in the environment.
1. Interaction with Biological Membranes
The perfluorinated nature of TDHA allows it to interact with lipid membranes. Studies indicate that such compounds can disrupt membrane integrity, leading to altered cellular functions. The hydrophobic interactions with lipid bilayers can affect the permeability of membranes, potentially impacting cellular signaling pathways and metabolic processes.
2. Fluorous Interactions in Drug Delivery
Recent research has highlighted the role of fluorous interactions in enhancing drug delivery systems. TDHA's unique properties enable it to serve as a carrier for drug molecules, improving their solubility and bioavailability. This has implications for developing new therapeutic strategies, particularly in targeting specific tissues or cells more effectively.
Case Study 1: Fluorous Proteomics
A study focused on the use of TDHA in fluorous proteomics demonstrated its ability to selectively bind fluorinated inhibitors in complex biological mixtures. This method improved the identification of protein targets by minimizing non-specific interactions during purification processes. The results indicated that compounds with longer fluorinated chains, like TDHA, showed superior binding affinity compared to shorter chains, enhancing the specificity of target identification .
Case Study 2: Environmental Impact and Bioaccumulation
Research on persistent organic pollutants has shown that compounds similar to TDHA can accumulate in biological systems. A comprehensive screening of industrial wastewater revealed that long-chain perfluorinated compounds exhibit significant bioaccumulation potential. This raises concerns about their environmental impact and potential health risks associated with exposure .
Research Findings
Recent studies have provided insights into the biological effects of TDHA:
- Toxicity Assessments : Animal models have shown that exposure to perfluorinated compounds can lead to various health issues, including hepatotoxicity and endocrine disruption. The specific effects of TDHA are still under investigation but are expected to follow similar patterns due to its structural characteristics .
- Bioactivity Profiles : Preliminary data suggest that TDHA may exhibit antimicrobial properties, although further research is needed to establish its efficacy against specific pathogens.
Comparative Analysis
Property | This compound | Other Perfluorinated Compounds |
---|---|---|
Chain Length | Long (C12) | Varies (C4-C14) |
Solubility | High in organic solvents | Generally high |
Membrane Interaction | Significant disruption | Varies |
Bioaccumulation Potential | High | Varies |
Toxicity | Under Investigation | Documented for several |
Q & A
Basic Research Questions
Q. What experimental methodologies are recommended for verifying the chemical structure of tridecafluoroheptanamide?
To confirm the structure, employ nuclear magnetic resonance (NMR) spectroscopy (¹H, ¹³C, and ¹⁹F), high-resolution mass spectrometry (HRMS), and X-ray crystallography. Cross-validate spectral data with reference standards from authoritative databases like the NIST Chemistry WebBook . For reproducibility, document solvent systems, acquisition parameters (e.g., pulse sequences for NMR), and calibration protocols for instruments.
Q. How should researchers design a controlled synthesis protocol for this compound to ensure purity and yield?
Use a stepwise approach:
- Optimize reaction conditions (temperature, solvent, catalyst) via Design of Experiments (DoE) to identify critical parameters.
- Monitor reaction progress with thin-layer chromatography (TLC) or in-situ FTIR.
- Purify the product using recrystallization or column chromatography, and validate purity via HPLC with UV detection (λ = 254 nm) .
- Report yields as mean ± standard deviation across triplicate trials.
Q. What analytical techniques are suitable for quantifying trace impurities in this compound samples?
Combine gas chromatography-mass spectrometry (GC-MS) for volatile impurities and liquid chromatography-tandem mass spectrometry (LC-MS/MS) for non-volatile residues. Calibrate instruments using certified reference materials and include recovery studies (spiked samples) to validate accuracy (85–115% recovery range) .
Advanced Research Questions
Q. How can researchers resolve contradictions in reported physicochemical properties (e.g., solubility, logP) of this compound across studies?
Conduct a systematic review:
- Compare experimental conditions (e.g., solvent polarity, temperature) and measurement techniques (e.g., shake-flask vs. chromatographic methods for logP).
- Perform meta-analysis using statistical tools (e.g., ANOVA) to assess variability sources.
- Replicate conflicting studies under standardized protocols and publish raw datasets with error margins .
Q. What strategies are effective for analyzing the environmental fate of this compound in multi-compartment systems (e.g., soil-water interfaces)?
Develop a tiered approach:
- Use laboratory-scale microcosms to simulate environmental matrices under controlled conditions (pH, organic matter content).
- Apply isotopic labeling (e.g., ¹³C) to track degradation pathways via LC-MS.
- Integrate computational models (e.g., quantitative structure-activity relationships, QSARs) to predict long-term bioaccumulation potential .
Q. How can researchers address challenges in reproducibility when scaling up this compound synthesis from lab to pilot-scale reactors?
Implement quality-by-design (QbD) principles:
- Characterize critical process parameters (CPPs) such as mixing efficiency and heat transfer coefficients using computational fluid dynamics (CFD).
- Validate scalability via batch consistency tests (e.g., ≤5% variability in yield/purity between lab and pilot batches).
- Document deviations using failure mode and effects analysis (FMEA) frameworks .
Q. What multidisciplinary approaches are recommended for studying this compound’s interactions with biological systems?
Combine in vitro and in silico methods:
- Perform cytotoxicity assays (e.g., MTT assay) with human cell lines, paired with molecular docking simulations to identify binding affinities.
- Use synchrotron-based X-ray absorption spectroscopy (XAS) to probe fluorine-protein interactions at atomic resolution.
- Publish negative results to mitigate publication bias .
Q. Methodological Guidance
Q. How should conflicting data on this compound’s thermal stability be addressed in peer-reviewed publications?
Adopt a transparency-focused methodology:
- Disclose all raw data (e.g., differential scanning calorimetry thermograms) in supplementary materials.
- Discuss potential confounding factors (e.g., sample hydration, heating rates).
- Propose a consensus protocol for future studies, emphasizing ISO/IEC 17025 accreditation for thermal analysis labs .
Q. What statistical frameworks are appropriate for analyzing dose-response relationships in this compound toxicity studies?
Use nonlinear regression models (e.g., Hill equation) to estimate EC₅₀ values.
- Validate assumptions via residual analysis and Kolmogorov-Smirnov tests for normality.
- Report confidence intervals (95% CI) and effect sizes (e.g., Cohen’s d) to contextualize biological significance .
Q. How can researchers ensure compliance with ethical and reporting standards when publishing this compound data?
Follow FAIR (Findable, Accessible, Interoperable, Reusable) principles:
Properties
IUPAC Name |
2,2,3,3,4,4,5,5,6,6,7,7,7-tridecafluoroheptanamide | |
---|---|---|
Source | PubChem | |
URL | https://pubchem.ncbi.nlm.nih.gov | |
Description | Data deposited in or computed by PubChem | |
InChI |
InChI=1S/C7H2F13NO/c8-2(9,1(21)22)3(10,11)4(12,13)5(14,15)6(16,17)7(18,19)20/h(H2,21,22) | |
Source | PubChem | |
URL | https://pubchem.ncbi.nlm.nih.gov | |
Description | Data deposited in or computed by PubChem | |
InChI Key |
SMJYTRJJHRJAED-UHFFFAOYSA-N | |
Source | PubChem | |
URL | https://pubchem.ncbi.nlm.nih.gov | |
Description | Data deposited in or computed by PubChem | |
Canonical SMILES |
C(=O)(C(C(C(C(C(C(F)(F)F)(F)F)(F)F)(F)F)(F)F)(F)F)N | |
Source | PubChem | |
URL | https://pubchem.ncbi.nlm.nih.gov | |
Description | Data deposited in or computed by PubChem | |
Molecular Formula |
C6F13C(O)NH2, C7H2F13NO | |
Record name | Heptanamide, 2,2,3,3,4,4,5,5,6,6,7,7,7-tridecafluoro- | |
Source | NORMAN Suspect List Exchange | |
Description | The NORMAN network enhances the exchange of information on emerging environmental substances, and encourages the validation and harmonisation of common measurement methods and monitoring tools so that the requirements of risk assessors and risk managers can be better met. The NORMAN Suspect List Exchange (NORMAN-SLE) is a central access point to find suspect lists relevant for various environmental monitoring questions, described in DOI:10.1186/s12302-022-00680-6 | |
Explanation | Data: CC-BY 4.0; Code (hosted by ECI, LCSB): Artistic-2.0 | |
Source | PubChem | |
URL | https://pubchem.ncbi.nlm.nih.gov | |
Description | Data deposited in or computed by PubChem | |
DSSTOX Substance ID |
DTXSID20377827 | |
Record name | Perfluoroheptanamide | |
Source | EPA DSSTox | |
URL | https://comptox.epa.gov/dashboard/DTXSID20377827 | |
Description | DSSTox provides a high quality public chemistry resource for supporting improved predictive toxicology. | |
Molecular Weight |
363.08 g/mol | |
Source | PubChem | |
URL | https://pubchem.ncbi.nlm.nih.gov | |
Description | Data deposited in or computed by PubChem | |
CAS No. |
2358-22-7 | |
Record name | Perfluoroheptanamide | |
Source | EPA DSSTox | |
URL | https://comptox.epa.gov/dashboard/DTXSID20377827 | |
Description | DSSTox provides a high quality public chemistry resource for supporting improved predictive toxicology. | |
Synthesis routes and methods I
Procedure details
Synthesis routes and methods II
Procedure details
Retrosynthesis Analysis
AI-Powered Synthesis Planning: Our tool employs the Template_relevance Pistachio, Template_relevance Bkms_metabolic, Template_relevance Pistachio_ringbreaker, Template_relevance Reaxys, Template_relevance Reaxys_biocatalysis model, leveraging a vast database of chemical reactions to predict feasible synthetic routes.
One-Step Synthesis Focus: Specifically designed for one-step synthesis, it provides concise and direct routes for your target compounds, streamlining the synthesis process.
Accurate Predictions: Utilizing the extensive PISTACHIO, BKMS_METABOLIC, PISTACHIO_RINGBREAKER, REAXYS, REAXYS_BIOCATALYSIS database, our tool offers high-accuracy predictions, reflecting the latest in chemical research and data.
Strategy Settings
Precursor scoring | Relevance Heuristic |
---|---|
Min. plausibility | 0.01 |
Model | Template_relevance |
Template Set | Pistachio/Bkms_metabolic/Pistachio_ringbreaker/Reaxys/Reaxys_biocatalysis |
Top-N result to add to graph | 6 |
Feasible Synthetic Routes
Disclaimer and Information on In-Vitro Research Products
Please be aware that all articles and product information presented on BenchChem are intended solely for informational purposes. The products available for purchase on BenchChem are specifically designed for in-vitro studies, which are conducted outside of living organisms. In-vitro studies, derived from the Latin term "in glass," involve experiments performed in controlled laboratory settings using cells or tissues. It is important to note that these products are not categorized as medicines or drugs, and they have not received approval from the FDA for the prevention, treatment, or cure of any medical condition, ailment, or disease. We must emphasize that any form of bodily introduction of these products into humans or animals is strictly prohibited by law. It is essential to adhere to these guidelines to ensure compliance with legal and ethical standards in research and experimentation.