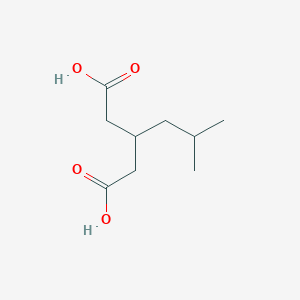
3-Isobutylglutaric acid
Overview
Description
Mechanism of Action
Target of Action
3-Isobutylglutaric acid is primarily used as an intermediate in the synthesis of (S)-Pregabalin . (S)-Pregabalin is a γ-amino butyric acid or (S)-3-isobutyl (GABA) analogue . It has been found to activate GAD (L-glutamic acid decarboxylase), promoting the production of GABA, one of the brain’s major inhibitory neurotransmitters .
Mode of Action
It is known that it plays a crucial role in the synthesis of (s)-pregabalin . (S)-Pregabalin has a dose-dependent protective effect on seizure and is a CNS-active compound . It is useful in anticonvulsant therapy due to its activation of GAD, which promotes the production of GABA .
Biochemical Pathways
The biochemical pathways affected by this compound are primarily related to its role in the synthesis of (S)-Pregabalin . By promoting the production of GABA, it influences the GABAergic system, which plays a crucial role in the central nervous system’s response to seizures .
Pharmacokinetics
It is known that it is used as an intermediate in the synthesis of (s)-pregabalin . The pharmacokinetic properties of (S)-Pregabalin, such as absorption, distribution, metabolism, and excretion, would be influenced by the properties of this compound.
Result of Action
The primary result of the action of this compound is the synthesis of (S)-Pregabalin . (S)-Pregabalin has analgesic, anticonvulsant, and anxiolytic activity . It is used in anticonvulsant therapy and has been found to have a dose-dependent protective effect on seizure .
Action Environment
The action environment of this compound is primarily within the biochemical pathways involved in the synthesis of (S)-Pregabalin . Environmental factors that could influence the compound’s action, efficacy, and stability include temperature, pH, and the presence of other compounds in the reaction mixture.
Preparation Methods
Synthetic Routes and Reaction Conditions
3-Isobutylglutaric acid can be synthesized through various methods. One common approach involves the reaction of isovaleraldehyde with a compound of formula II in the presence of a non-polar organic solvent . Another method involves the esterification of butanol with glutaric acid under appropriate conditions .
Industrial Production Methods
In industrial settings, this compound is often produced as an intermediate in the synthesis of pregabalin. The process typically involves the use of advanced chemical synthesis techniques to ensure high purity and yield .
Chemical Reactions Analysis
Types of Reactions
3-Isobutylglutaric acid undergoes various chemical reactions, including:
Oxidation: It can be oxidized to form corresponding ketones or aldehydes.
Reduction: It can be reduced to form alcohols.
Substitution: It can undergo substitution reactions to form various derivatives.
Common Reagents and Conditions
Oxidation: Common oxidizing agents include potassium permanganate and chromium trioxide.
Reduction: Reducing agents such as lithium aluminum hydride and sodium borohydride are often used.
Substitution: Conditions vary depending on the desired product but often involve the use of catalysts and specific solvents.
Major Products Formed
Oxidation: Ketones and aldehydes.
Reduction: Alcohols.
Substitution: Various derivatives depending on the substituent introduced.
Scientific Research Applications
3-Isobutylglutaric acid has several scientific research applications:
Chemistry: It is used as an intermediate in the synthesis of various organic compounds.
Biology: It is studied for its potential biological activities and interactions with enzymes.
Industry: It is used in the production of pharmaceuticals and other fine chemicals.
Comparison with Similar Compounds
Similar Compounds
3-Butylglutaric acid: Another intermediate in the synthesis of pregabalin.
3-Isobutylpentanedioic acid: A structural isomer with similar properties.
Uniqueness
3-Isobutylglutaric acid is unique due to its specific structure, which makes it an essential intermediate in the synthesis of pregabalin. Its ability to undergo various chemical reactions and form different derivatives also adds to its versatility and importance in scientific research and industrial applications .
Properties
IUPAC Name |
3-(2-methylpropyl)pentanedioic acid | |
---|---|---|
Source | PubChem | |
URL | https://pubchem.ncbi.nlm.nih.gov | |
Description | Data deposited in or computed by PubChem | |
InChI |
InChI=1S/C9H16O4/c1-6(2)3-7(4-8(10)11)5-9(12)13/h6-7H,3-5H2,1-2H3,(H,10,11)(H,12,13) | |
Source | PubChem | |
URL | https://pubchem.ncbi.nlm.nih.gov | |
Description | Data deposited in or computed by PubChem | |
InChI Key |
UATSLDZQNXAKMA-UHFFFAOYSA-N | |
Source | PubChem | |
URL | https://pubchem.ncbi.nlm.nih.gov | |
Description | Data deposited in or computed by PubChem | |
Canonical SMILES |
CC(C)CC(CC(=O)O)CC(=O)O | |
Source | PubChem | |
URL | https://pubchem.ncbi.nlm.nih.gov | |
Description | Data deposited in or computed by PubChem | |
Molecular Formula |
C9H16O4 | |
Source | PubChem | |
URL | https://pubchem.ncbi.nlm.nih.gov | |
Description | Data deposited in or computed by PubChem | |
DSSTOX Substance ID |
DTXSID00450420 | |
Record name | 3-isobutylglutaric acid | |
Source | EPA DSSTox | |
URL | https://comptox.epa.gov/dashboard/DTXSID00450420 | |
Description | DSSTox provides a high quality public chemistry resource for supporting improved predictive toxicology. | |
Molecular Weight |
188.22 g/mol | |
Source | PubChem | |
URL | https://pubchem.ncbi.nlm.nih.gov | |
Description | Data deposited in or computed by PubChem | |
CAS No. |
75143-89-4 | |
Record name | 3-Isobutylglutaric acid | |
Source | CAS Common Chemistry | |
URL | https://commonchemistry.cas.org/detail?cas_rn=75143-89-4 | |
Description | CAS Common Chemistry is an open community resource for accessing chemical information. Nearly 500,000 chemical substances from CAS REGISTRY cover areas of community interest, including common and frequently regulated chemicals, and those relevant to high school and undergraduate chemistry classes. This chemical information, curated by our expert scientists, is provided in alignment with our mission as a division of the American Chemical Society. | |
Explanation | The data from CAS Common Chemistry is provided under a CC-BY-NC 4.0 license, unless otherwise stated. | |
Record name | Isobutylglutaric acid | |
Source | ChemIDplus | |
URL | https://pubchem.ncbi.nlm.nih.gov/substance/?source=chemidplus&sourceid=0075143894 | |
Description | ChemIDplus is a free, web search system that provides access to the structure and nomenclature authority files used for the identification of chemical substances cited in National Library of Medicine (NLM) databases, including the TOXNET system. | |
Record name | 3-isobutylglutaric acid | |
Source | EPA DSSTox | |
URL | https://comptox.epa.gov/dashboard/DTXSID00450420 | |
Description | DSSTox provides a high quality public chemistry resource for supporting improved predictive toxicology. | |
Record name | 3-(2-Methylpropyl)pentanedioic Acid | |
Source | European Chemicals Agency (ECHA) | |
URL | https://echa.europa.eu/information-on-chemicals | |
Description | The European Chemicals Agency (ECHA) is an agency of the European Union which is the driving force among regulatory authorities in implementing the EU's groundbreaking chemicals legislation for the benefit of human health and the environment as well as for innovation and competitiveness. | |
Explanation | Use of the information, documents and data from the ECHA website is subject to the terms and conditions of this Legal Notice, and subject to other binding limitations provided for under applicable law, the information, documents and data made available on the ECHA website may be reproduced, distributed and/or used, totally or in part, for non-commercial purposes provided that ECHA is acknowledged as the source: "Source: European Chemicals Agency, http://echa.europa.eu/". Such acknowledgement must be included in each copy of the material. ECHA permits and encourages organisations and individuals to create links to the ECHA website under the following cumulative conditions: Links can only be made to webpages that provide a link to the Legal Notice page. | |
Record name | ISOBUTYLGLUTARIC ACID | |
Source | FDA Global Substance Registration System (GSRS) | |
URL | https://gsrs.ncats.nih.gov/ginas/app/beta/substances/EMH1IVR5QH | |
Description | The FDA Global Substance Registration System (GSRS) enables the efficient and accurate exchange of information on what substances are in regulated products. Instead of relying on names, which vary across regulatory domains, countries, and regions, the GSRS knowledge base makes it possible for substances to be defined by standardized, scientific descriptions. | |
Explanation | Unless otherwise noted, the contents of the FDA website (www.fda.gov), both text and graphics, are not copyrighted. They are in the public domain and may be republished, reprinted and otherwise used freely by anyone without the need to obtain permission from FDA. Credit to the U.S. Food and Drug Administration as the source is appreciated but not required. | |
Synthesis routes and methods
Procedure details
Retrosynthesis Analysis
AI-Powered Synthesis Planning: Our tool employs the Template_relevance Pistachio, Template_relevance Bkms_metabolic, Template_relevance Pistachio_ringbreaker, Template_relevance Reaxys, Template_relevance Reaxys_biocatalysis model, leveraging a vast database of chemical reactions to predict feasible synthetic routes.
One-Step Synthesis Focus: Specifically designed for one-step synthesis, it provides concise and direct routes for your target compounds, streamlining the synthesis process.
Accurate Predictions: Utilizing the extensive PISTACHIO, BKMS_METABOLIC, PISTACHIO_RINGBREAKER, REAXYS, REAXYS_BIOCATALYSIS database, our tool offers high-accuracy predictions, reflecting the latest in chemical research and data.
Strategy Settings
Precursor scoring | Relevance Heuristic |
---|---|
Min. plausibility | 0.01 |
Model | Template_relevance |
Template Set | Pistachio/Bkms_metabolic/Pistachio_ringbreaker/Reaxys/Reaxys_biocatalysis |
Top-N result to add to graph | 6 |
Feasible Synthetic Routes
Q1: What is the primary synthetic route for 3-Isobutylglutaric acid?
A1: this compound is typically synthesized through a multi-step process starting with isovaleraldehyde. The process involves a Knoevenagel condensation with ethyl cyanoacetate, followed by a Michael addition with diethyl malonate. Subsequent hydrolysis and decarboxylation steps yield the desired this compound. [, , ] This route has proven to be efficient and adaptable for industrial-scale production.
Q2: What is the role of this compound in the synthesis of Pregabalin?
A2: this compound acts as a crucial precursor in the synthesis of Pregabalin. [] It undergoes several transformations, including conversion to its anhydride form, ammonolysis to form 3-(carbamoylmethyl)-5-methylhexanoic acid, and finally, a Hofmann rearrangement to yield 3-aminomethyl-5-methylhexanoic acid (the racemic precursor to Pregabalin). [, ]
Q3: Are there alternative synthetic pathways for this compound?
A3: Yes, an alternative method utilizes Malonamide nitrile and isovaleraldehyde as starting materials. [] This method employs base-catalyzed condensation under mild conditions, followed by hydrolysis in acidic conditions to obtain this compound. This approach boasts high yields and a reduced environmental footprint compared to traditional methods.
Q4: What impurities are commonly encountered during the synthesis of this compound?
A4: During the synthesis of Pregabalin, several impurities related to this compound have been identified. These include the unreacted acid itself, its (R)-enantiomer in both the acid and amide forms, and novel impurities like the isobutyl and isopropyl esters of (S)-3-aminomethyl-5-methylhexanoic acid. [] Understanding and controlling these impurities is essential for ensuring the quality and safety of Pregabalin.
Disclaimer and Information on In-Vitro Research Products
Please be aware that all articles and product information presented on BenchChem are intended solely for informational purposes. The products available for purchase on BenchChem are specifically designed for in-vitro studies, which are conducted outside of living organisms. In-vitro studies, derived from the Latin term "in glass," involve experiments performed in controlled laboratory settings using cells or tissues. It is important to note that these products are not categorized as medicines or drugs, and they have not received approval from the FDA for the prevention, treatment, or cure of any medical condition, ailment, or disease. We must emphasize that any form of bodily introduction of these products into humans or animals is strictly prohibited by law. It is essential to adhere to these guidelines to ensure compliance with legal and ethical standards in research and experimentation.