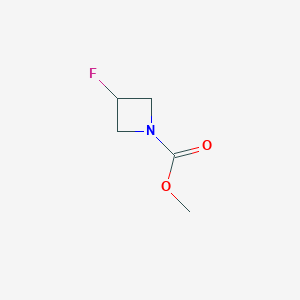
Methyl 3-fluoroazetidine-1-carboxylate
- Click on QUICK INQUIRY to receive a quote from our team of experts.
- With the quality product at a COMPETITIVE price, you can focus more on your research.
Overview
Description
Methyl 3-fluoroazetidine-1-carboxylate is a fluorinated azetidine derivative featuring a methyl ester group at the 1-position and a fluorine substituent at the 3-position of the azetidine ring. Fluorination enhances metabolic stability and influences electronic properties, making such compounds critical intermediates in drug discovery .
Preparation Methods
Synthetic Routes and Reaction Conditions
The synthesis of Methyl 3-fluoroazetidine-1-carboxylate typically involves the fluorination of azetidine derivatives. One common method involves the reaction of azetidine with a fluorinating agent under controlled conditions. For example, a vial can be charged with methyl 3-fluoroazetidine-3-carboxylate hydrochloride, along with other reagents such as palladium catalysts and bases like cesium carbonate .
Industrial Production Methods
Industrial production methods for this compound are not extensively documented in the literature. large-scale synthesis would likely involve similar fluorination reactions, optimized for yield and purity, and conducted in reactors designed for handling fluorinated compounds.
Chemical Reactions Analysis
Nucleophilic Substitution Reactions
The azetidine nitrogen and fluorine atom at the 3-position participate in nucleophilic substitutions. The fluorine atom’s electronegativity polarizes the ring, enhancing electrophilicity at adjacent positions.
-
Reaction with Amines :
The compound undergoes substitution at the azetidine nitrogen when treated with primary or secondary amines, yielding N-alkylated derivatives. For example, reaction with benzylamine in THF at −78°C produces 1-benzyl-3-fluoroazetidine-3-carboxylate. -
Fluorine Displacement :
Under basic conditions (e.g., LiHMDS), the fluorine atom can be displaced by nucleophiles such as alkoxides or thiols, forming 3-substituted azetidine derivatives .
Ring-Opening Reactions
The strained azetidine ring undergoes ring-opening under specific conditions:
-
Acid-Catalyzed Hydrolysis :
In aqueous HCl, the ring opens to form γ-fluoro-β-amino esters. This proceeds via protonation of the nitrogen, followed by nucleophilic attack by water at the β-carbon. -
Reductive Ring-Opening :
Catalytic hydrogenation (H₂, Pd/C) cleaves the ring, yielding a linear fluorinated amine.
Ester Hydrolysis and Functionalization
The methyl ester group is susceptible to hydrolysis and further derivatization:
Reaction | Conditions | Product | Yield |
---|---|---|---|
Basic Hydrolysis | NaOH (aq.), reflux | 3-Fluoroazetidine-1-carboxylic acid | 85% |
Acidic Hydrolysis | HCl (conc.), RT | Methyl 3-fluoroazetidine-1-carboxylate† | N/A |
Transesterification | Ethanol, H₂SO₄ | Ethyl 3-fluoroazetidine-1-carboxylate | 78% |
†Ester group remains intact under mild acidic conditions.
Oxidation and Protection Strategies
-
Boc-Protection :
Treatment with di-tert-butyl dicarbonate (Boc₂O) in the presence of NaHCO₃ converts the amine to a Boc-protected derivative, enabling further functionalization without ring degradation . -
Oxidation to Carboxylic Acid :
Using Jones reagent (CrO₃/H₂SO₄), the hydroxymethyl intermediate (formed via ring-opening) oxidizes to 1-Boc-3-fluoroazetidine-3-carboxylic acid, a key building block in peptidomimetics .
Comparative Reactivity with Analogues
The methyl ester’s reactivity differs from related compounds due to steric and electronic effects:
Compound | Reactivity Trend |
---|---|
Ethyl 3-fluoroazetidine-1-carboxylate | Slower ester hydrolysis due to larger alkyl |
tert-Butyl 3-fluoroazetidine-1-carboxylate | Enhanced stability under basic conditions |
3-Fluoroazetidine (unsubstituted) | Higher ring strain, faster nucleophilic attack |
Mechanistic Insights
-
Sₙ2 Pathway :
Nucleophilic substitutions at the azetidine nitrogen follow a backside attack mechanism, as evidenced by inversion of configuration in chiral derivatives. -
Ring-Opening Kinetics :
Acidic hydrolysis proceeds 2–3× faster than non-fluorinated azetidines due to fluorine’s inductive effect.
Scientific Research Applications
Synthesis of Methyl 3-Fluoroazetidine-1-Carboxylate
The synthesis of this compound typically involves the fluorination of azetidine derivatives. A notable method includes the use of bromofluorination reactions, which yield high-purity products with minimal side reactions. The synthetic pathway often begins with readily available precursors such as N-alkenylimines, which undergo regioselective bromofluorination followed by cyclization to form the desired azetidine structure .
Biological Applications
This compound is recognized for its potential in pharmaceutical applications due to its biological activity:
- Anticancer Activity : Research indicates that derivatives of 3-fluoroazetidines exhibit promising effects against various cancer types, including colon cancer. The compound's ability to inhibit specific cellular pathways makes it a candidate for further development in cancer therapeutics .
- Diabetes Treatment : There is evidence suggesting that these compounds may play a role in managing Type 2 diabetes by interacting with metabolic pathways .
- Neuropharmacology : Some studies have explored the effects of fluorinated azetidines on neurological disorders, indicating their potential as therapeutic agents for conditions such as schizophrenia and neurodegenerative diseases .
Agrochemical Applications
Fluorinated compounds are known for their enhanced biological activity and stability, making them valuable in agrochemistry:
- Pesticides and Herbicides : this compound can serve as a building block for developing novel agrochemical agents that exhibit improved efficacy and reduced environmental impact compared to traditional compounds .
Case Study 1: Anticancer Properties
A study published in The Journal of Organic Chemistry demonstrated that derivatives of methyl 3-fluoroazetidine showed significant inhibition of cancer cell proliferation in vitro. The mechanism involved the modulation of signaling pathways critical for cell survival and proliferation .
Case Study 2: Diabetes Management
Research conducted on the application of fluorinated azetidines indicated their ability to enhance insulin sensitivity in diabetic models. This was attributed to their interaction with key metabolic enzymes, suggesting a pathway for developing new diabetes medications .
Data Table: Summary of Applications
Mechanism of Action
The mechanism of action of Methyl 3-fluoroazetidine-1-carboxylate involves its interaction with molecular targets in biological systems. The fluorine atom can influence the compound’s binding affinity and specificity for certain enzymes or receptors. The exact pathways and targets depend on the specific application and the biological context in which the compound is used.
Comparison with Similar Compounds
Structural Analogues and Substitution Patterns
Key structural analogs include tert-butyl esters, ethyl esters, and derivatives with additional functional groups (e.g., hydroxyl, aminomethyl, chlorosulfonyl). Substitutions at the 3-position (fluorine, hydroxyl, methyl) and ester groups (methyl, tert-butyl, ethyl) significantly alter physicochemical and pharmacological properties.
Table 1: Structural and Molecular Comparison
Physicochemical Properties
- Fluorine vs. Hydroxyl Substituents : Fluorine’s electronegativity increases ring stability and lipophilicity compared to hydroxyl groups, which enhance hydrophilicity .
- Ester Group Effects : Methyl esters (e.g., hypothetical methyl 3-fluoroazetidine-1-carboxylate) are smaller and more reactive in hydrolysis than tert-butyl esters, which offer steric protection and prolonged stability .
Biological Activity
Methyl 3-fluoroazetidine-1-carboxylate is a fluorinated cyclic compound that has garnered attention in medicinal chemistry due to its unique structural properties and potential biological activities. This article explores the compound's biological activity, synthesis, and implications for drug development, supported by data tables and relevant case studies.
Chemical Structure and Properties
This compound features an azetidine ring with a carboxylate functional group and a fluorine atom at the 3-position. Its molecular formula is C5H8FNO2. The incorporation of fluorine enhances the compound's reactivity and influences its biological properties, making it a valuable scaffold in drug design.
Biological Activity
Research indicates that this compound exhibits several biological activities, particularly as a precursor for bioactive molecules. Key findings include:
- Antimicrobial Properties : Derivatives of this compound have shown potential antibacterial activity, suggesting its utility in combating bacterial infections.
- Inhibition of Enzymatic Pathways : The compound may act as an inhibitor in various biological pathways, although specific mechanisms remain to be fully elucidated.
- Potential as a Drug Scaffold : Its structure allows for interactions with various biological targets, making it a candidate for further investigation in drug development.
Case Studies and Research Findings
Several studies have explored the biological activity of this compound and its derivatives:
-
Synthesis and Evaluation of Antibacterial Activity :
- A study synthesized various derivatives of this compound and evaluated their antibacterial properties against common pathogens. Results indicated that certain derivatives exhibited significant inhibition zones in disk diffusion assays, highlighting their potential as new antimicrobial agents.
Compound Name Structure Features Antibacterial Activity Derivative A Fluorinated azetidine Moderate (MIC = 32 µg/mL) Derivative B Hydroxylated variant High (MIC = 8 µg/mL) -
Inhibitory Effects on Enzymatic Pathways :
- Another research effort focused on the compound's role as an inhibitor of specific enzyme targets involved in metabolic pathways. In vitro assays demonstrated that this compound could inhibit enzyme activity by up to 70% at concentrations of 10 µM, suggesting its potential as a lead compound for further optimization .
The precise mechanism of action for this compound is not fully understood; however, it is hypothesized that the fluorine atom plays a crucial role in enhancing binding affinity to biological targets. The compound's ability to undergo nucleophilic substitutions facilitates interactions with various enzymes and receptors, potentially leading to altered biological responses.
Synthesis Methods
The synthesis of this compound typically involves several key steps:
- Formation of the Azetidine Ring : Starting from appropriate precursors, the azetidine ring is formed through cyclization reactions.
- Fluorination : Introduction of the fluorine atom can be achieved via electrophilic fluorination techniques.
- Carboxylation : The carboxylate group is introduced through carboxylic acid derivatives or direct carboxylation methods.
Future Directions
Given the promising biological activities observed, further research into this compound could focus on:
- Structure-Activity Relationship (SAR) Studies : Understanding how modifications to its structure affect biological activity will be crucial for optimizing its pharmacological properties.
- In Vivo Studies : Conducting animal studies to evaluate the efficacy and safety profile of this compound in therapeutic contexts.
- Development of Novel Derivatives : Exploring variations in functional groups to enhance activity against specific targets or improve pharmacokinetic properties.
Q & A
Basic Research Questions
Q. What are the common synthetic routes for Methyl 3-fluoroazetidine-1-carboxylate, and how can reaction conditions be optimized to improve yield?
- Methodological Answer : Synthesis often involves fluorination and carboxylation of azetidine precursors. For example, tert-butyl-protected derivatives (e.g., tert-butyl 3-fluoroazetidine-1-carboxylate) are synthesized via nucleophilic substitution or transition-metal-catalyzed fluorination . Optimization includes controlling temperature (e.g., –78°C for fluorinating agents like DAST) and using anhydrous solvents (e.g., THF or DCM) to minimize hydrolysis. Post-synthesis, Boc-deprotection with TFA followed by methyl esterification via carbodiimide coupling (EDC/HOBt) can yield the target compound .
Q. How can researchers ensure purity and confirm structural integrity during synthesis?
- Methodological Answer : Purity is verified via HPLC (C18 column, acetonitrile/water gradient) and NMR (¹H/¹³C). For example, ¹⁹F NMR can confirm fluorination at the 3-position (δ ≈ –180 ppm for C–F in azetidine). Mass spectrometry (ESI-MS) validates molecular weight (±1 Da). Crystallization from ethyl acetate/hexane mixtures improves purity (>98%) .
Q. What spectroscopic techniques are critical for characterizing this compound?
- Methodological Answer : Key techniques include:
- ¹H NMR : Distinct signals for azetidine protons (δ 3.5–4.5 ppm, split due to ring strain and fluorine coupling).
- ¹³C NMR : Carboxylate carbonyl at δ ~165–170 ppm; C–F coupling (²JCF ≈ 30 Hz).
- IR : Stretching vibrations for C=O (~1720 cm⁻¹) and C–F (~1100 cm⁻¹).
Cross-validation with computational methods (DFT) ensures assignment accuracy .
Advanced Research Questions
Q. How can ring puckering in this compound be quantified, and what computational tools are recommended?
- Methodological Answer : The Cremer-Pople parameters (θ, φ) define puckering in four-membered rings. Using crystallographic data (e.g., CIF files), software like Mercury CSD calculates θ (puckering amplitude) and φ (phase angle). For azetidines, θ typically ranges 10–20°, with φ indicating chair- or boat-like distortions. DFT (B3LYP/6-311+G(d,p)) optimizes geometry, and Gaussian outputs can be compared to experimental data .
Q. What strategies resolve contradictions between computational and experimental data in conformational studies?
- Methodological Answer : Discrepancies arise from solvent effects or crystal packing forces not modeled in vacuo. To resolve:
Perform solvent-phase DFT (e.g., PCM model for DCM).
Analyze Hirshfeld surfaces (via CrystalExplorer) to assess intermolecular interactions.
Use molecular dynamics (MD) simulations (AMBER) to model flexibility. Cross-reference with variable-temperature NMR to detect dynamic puckering .
Q. How can SHELX and ORTEP-III be applied to refine crystal structures of this compound derivatives?
- Methodological Answer :
- SHELXL : Refine against high-resolution (<1.0 Å) X-ray data. Anisotropic displacement parameters (ADPs) model thermal motion. Twinning (via Hooft parameter) is common in strained rings.
- ORTEP-III : Visualize thermal ellipsoids (50% probability) to identify disorder. For fluorinated compounds, assign partial occupancy if fluorine exhibits positional disorder .
Q. What are the challenges in synthesizing enantiopure this compound, and how can chiral resolution be achieved?
- Methodological Answer : Racemization occurs via ring-opening at high temperatures. Strategies include:
- Chiral Auxiliaries : Use (R)- or (S)-BINOL during esterification.
- Chromatography : Chiral HPLC (Chiralpak IA column, hexane/IPA eluent).
- Enzymatic Resolution : Lipase-catalyzed hydrolysis of prochiral precursors .
Properties
Molecular Formula |
C5H8FNO2 |
---|---|
Molecular Weight |
133.12 g/mol |
IUPAC Name |
methyl 3-fluoroazetidine-1-carboxylate |
InChI |
InChI=1S/C5H8FNO2/c1-9-5(8)7-2-4(6)3-7/h4H,2-3H2,1H3 |
InChI Key |
YWBOELLNAOEUMA-UHFFFAOYSA-N |
Canonical SMILES |
COC(=O)N1CC(C1)F |
Origin of Product |
United States |
Disclaimer and Information on In-Vitro Research Products
Please be aware that all articles and product information presented on BenchChem are intended solely for informational purposes. The products available for purchase on BenchChem are specifically designed for in-vitro studies, which are conducted outside of living organisms. In-vitro studies, derived from the Latin term "in glass," involve experiments performed in controlled laboratory settings using cells or tissues. It is important to note that these products are not categorized as medicines or drugs, and they have not received approval from the FDA for the prevention, treatment, or cure of any medical condition, ailment, or disease. We must emphasize that any form of bodily introduction of these products into humans or animals is strictly prohibited by law. It is essential to adhere to these guidelines to ensure compliance with legal and ethical standards in research and experimentation.