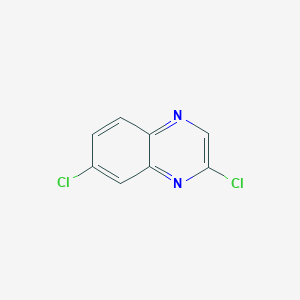
2,7-Dichloroquinoxaline
Overview
Description
2,7-Dichloroquinoxaline is a nitrogen-containing heterocyclic compound with the molecular formula C8H4Cl2N2. It belongs to the quinoxaline family, which is characterized by a bicyclic structure consisting of fused benzene and pyrazine rings.
Mechanism of Action
Target of Action
2,7-Dichloroquinoxaline is a derivative of quinoxaline, a class of nitrogen-based heterocyclic compounds . Quinoxaline derivatives have been found to have several prominent pharmacological effects, including antifungal, antibacterial, antiviral, and antimicrobial properties . .
Mode of Action
Quinoxaline derivatives, in general, have been found to interact with various targets, receptors, or microorganisms
Biochemical Pathways
Quinoxaline derivatives have been found to have a wide range of physicochemical and biological activities , suggesting that they may affect multiple biochemical pathways
Result of Action
Quinoxaline derivatives have been found to have a wide range of biological properties , suggesting that they may have various molecular and cellular effects.
Biochemical Analysis
Biochemical Properties
2,7-Dichloroquinoxaline plays a significant role in biochemical reactions, particularly in the inhibition of certain enzymes. It interacts with enzymes such as tyrosine kinases and phosphatases, which are crucial in cell signaling pathways. The compound binds to the active sites of these enzymes, inhibiting their activity and thereby affecting downstream signaling processes. Additionally, this compound has been shown to interact with proteins involved in DNA repair mechanisms, further highlighting its importance in cellular biochemistry .
Cellular Effects
The effects of this compound on various cell types and cellular processes are profound. It has been observed to influence cell function by modulating cell signaling pathways, gene expression, and cellular metabolism. For instance, in cancer cells, this compound can induce apoptosis by increasing reactive oxygen species (ROS) levels and inhibiting hypoxia-inducible factor-1 alpha (HIF-1α) pathways . This leads to reduced cell proliferation and metastasis. Moreover, the compound affects gene expression by downregulating genes involved in cell survival and upregulating those associated with cell death .
Molecular Mechanism
At the molecular level, this compound exerts its effects through several mechanisms. It binds to the active sites of enzymes, inhibiting their catalytic activity. This inhibition can lead to the accumulation of substrates and the depletion of products, thereby disrupting normal cellular functions. Additionally, this compound can induce conformational changes in proteins, affecting their stability and function. The compound also influences gene expression by interacting with transcription factors and other regulatory proteins, leading to changes in the transcriptional landscape of the cell .
Temporal Effects in Laboratory Settings
In laboratory settings, the effects of this compound can change over time. The compound is relatively stable under standard storage conditions, but its activity can degrade over extended periods. In in vitro studies, the effects of this compound on cellular function can be observed within hours of treatment, with significant changes in cell viability and gene expression occurring within 24 to 48 hours. Long-term studies have shown that prolonged exposure to this compound can lead to sustained inhibition of enzyme activity and persistent changes in cellular metabolism .
Dosage Effects in Animal Models
The effects of this compound vary with different dosages in animal models. At low doses, the compound can effectively inhibit target enzymes without causing significant toxicity. At higher doses, this compound can induce toxic effects, including liver and kidney damage. Threshold effects have been observed, where a certain dosage is required to achieve therapeutic effects, but exceeding this threshold can lead to adverse outcomes. These findings highlight the importance of dosage optimization in the therapeutic application of this compound .
Metabolic Pathways
This compound is involved in several metabolic pathways, primarily those related to its biotransformation and elimination. The compound is metabolized by liver enzymes, including cytochrome P450 enzymes, which convert it into more water-soluble metabolites for excretion. This metabolic process can affect the compound’s bioavailability and efficacy. Additionally, this compound can influence metabolic flux by altering the levels of key metabolites and intermediates in various biochemical pathways .
Transport and Distribution
Within cells and tissues, this compound is transported and distributed through passive diffusion and active transport mechanisms. The compound can interact with transporters and binding proteins, which facilitate its movement across cellular membranes and its accumulation in specific cellular compartments. This distribution pattern can affect the compound’s localization and its overall biological activity .
Subcellular Localization
The subcellular localization of this compound is critical for its activity and function. The compound is primarily localized in the cytoplasm, where it interacts with various enzymes and proteins. It can also be found in the nucleus, where it affects gene expression and DNA repair processes. The localization of this compound is influenced by targeting signals and post-translational modifications, which direct it to specific cellular compartments .
Preparation Methods
Synthetic Routes and Reaction Conditions: The synthesis of 2,7-Dichloroquinoxaline typically involves the condensation reaction between ortho-phenylenediamine and dicarbonyl compounds under specific conditions. One common method is the reaction of 2,7-dichloro-1,4-benzoquinone with ortho-phenylenediamine in the presence of a suitable catalyst. This reaction is usually carried out in an organic solvent such as ethanol or acetonitrile at elevated temperatures .
Industrial Production Methods: Industrial production of this compound often employs similar synthetic routes but on a larger scale. The process involves optimizing reaction conditions to ensure high yield and purity. Catalysts and solvents are chosen based on cost-effectiveness and environmental considerations. Green chemistry approaches are increasingly being adopted to minimize the environmental impact of the synthesis .
Chemical Reactions Analysis
Types of Reactions: 2,7-Dichloroquinoxaline undergoes various chemical reactions, including:
Substitution Reactions: The chlorine atoms can be substituted with different nucleophiles, such as amines or thiols, to form a variety of derivatives.
Oxidation and Reduction Reactions: The compound can be oxidized or reduced under specific conditions to yield different products.
Common Reagents and Conditions:
Substitution Reactions: Common reagents include amines, thiols, and other nucleophiles.
Oxidation and Reduction Reactions: Reagents such as hydrogen peroxide or sodium borohydride are used for oxidation and reduction, respectively.
Major Products Formed:
Substitution Products: Various 2,7-disubstituted quinoxalines.
Oxidation and Reduction Products: Different quinoxaline derivatives depending on the specific reaction conditions.
Scientific Research Applications
2,7-Dichloroquinoxaline has a wide range of applications in scientific research:
Comparison with Similar Compounds
- 2,3-Dichloroquinoxaline
- 6,7-Dichloroquinoxaline
- 2,3-Dichloroquinoxaline-2,3-dione
Comparison: 2,7-Dichloroquinoxaline is unique due to its specific substitution pattern, which imparts distinct chemical and biological properties. Compared to other dichloroquinoxalines, it may exhibit different reactivity and potency in various applications .
Properties
IUPAC Name |
2,7-dichloroquinoxaline | |
---|---|---|
Source | PubChem | |
URL | https://pubchem.ncbi.nlm.nih.gov | |
Description | Data deposited in or computed by PubChem | |
InChI |
InChI=1S/C8H4Cl2N2/c9-5-1-2-6-7(3-5)12-8(10)4-11-6/h1-4H | |
Source | PubChem | |
URL | https://pubchem.ncbi.nlm.nih.gov | |
Description | Data deposited in or computed by PubChem | |
InChI Key |
YNASAKVGUCRNEP-UHFFFAOYSA-N | |
Source | PubChem | |
URL | https://pubchem.ncbi.nlm.nih.gov | |
Description | Data deposited in or computed by PubChem | |
Canonical SMILES |
C1=CC2=NC=C(N=C2C=C1Cl)Cl | |
Source | PubChem | |
URL | https://pubchem.ncbi.nlm.nih.gov | |
Description | Data deposited in or computed by PubChem | |
Molecular Formula |
C8H4Cl2N2 | |
Source | PubChem | |
URL | https://pubchem.ncbi.nlm.nih.gov | |
Description | Data deposited in or computed by PubChem | |
DSSTOX Substance ID |
DTXSID30374226 | |
Record name | 2,7-Dichloroquinoxaline | |
Source | EPA DSSTox | |
URL | https://comptox.epa.gov/dashboard/DTXSID30374226 | |
Description | DSSTox provides a high quality public chemistry resource for supporting improved predictive toxicology. | |
Molecular Weight |
199.03 g/mol | |
Source | PubChem | |
URL | https://pubchem.ncbi.nlm.nih.gov | |
Description | Data deposited in or computed by PubChem | |
CAS No. |
59489-31-5 | |
Record name | 2,7-Dichloroquinoxaline | |
Source | CAS Common Chemistry | |
URL | https://commonchemistry.cas.org/detail?cas_rn=59489-31-5 | |
Description | CAS Common Chemistry is an open community resource for accessing chemical information. Nearly 500,000 chemical substances from CAS REGISTRY cover areas of community interest, including common and frequently regulated chemicals, and those relevant to high school and undergraduate chemistry classes. This chemical information, curated by our expert scientists, is provided in alignment with our mission as a division of the American Chemical Society. | |
Explanation | The data from CAS Common Chemistry is provided under a CC-BY-NC 4.0 license, unless otherwise stated. | |
Record name | 2,7-Dichloroquinoxaline | |
Source | EPA DSSTox | |
URL | https://comptox.epa.gov/dashboard/DTXSID30374226 | |
Description | DSSTox provides a high quality public chemistry resource for supporting improved predictive toxicology. | |
Record name | 59489-31-5 | |
Source | European Chemicals Agency (ECHA) | |
URL | https://echa.europa.eu/information-on-chemicals | |
Description | The European Chemicals Agency (ECHA) is an agency of the European Union which is the driving force among regulatory authorities in implementing the EU's groundbreaking chemicals legislation for the benefit of human health and the environment as well as for innovation and competitiveness. | |
Explanation | Use of the information, documents and data from the ECHA website is subject to the terms and conditions of this Legal Notice, and subject to other binding limitations provided for under applicable law, the information, documents and data made available on the ECHA website may be reproduced, distributed and/or used, totally or in part, for non-commercial purposes provided that ECHA is acknowledged as the source: "Source: European Chemicals Agency, http://echa.europa.eu/". Such acknowledgement must be included in each copy of the material. ECHA permits and encourages organisations and individuals to create links to the ECHA website under the following cumulative conditions: Links can only be made to webpages that provide a link to the Legal Notice page. | |
Retrosynthesis Analysis
AI-Powered Synthesis Planning: Our tool employs the Template_relevance Pistachio, Template_relevance Bkms_metabolic, Template_relevance Pistachio_ringbreaker, Template_relevance Reaxys, Template_relevance Reaxys_biocatalysis model, leveraging a vast database of chemical reactions to predict feasible synthetic routes.
One-Step Synthesis Focus: Specifically designed for one-step synthesis, it provides concise and direct routes for your target compounds, streamlining the synthesis process.
Accurate Predictions: Utilizing the extensive PISTACHIO, BKMS_METABOLIC, PISTACHIO_RINGBREAKER, REAXYS, REAXYS_BIOCATALYSIS database, our tool offers high-accuracy predictions, reflecting the latest in chemical research and data.
Strategy Settings
Precursor scoring | Relevance Heuristic |
---|---|
Min. plausibility | 0.01 |
Model | Template_relevance |
Template Set | Pistachio/Bkms_metabolic/Pistachio_ringbreaker/Reaxys/Reaxys_biocatalysis |
Top-N result to add to graph | 6 |
Feasible Synthetic Routes
Q1: What is the significance of 2,7-Dichloroquinoxaline in antitumor research?
A: this compound serves as a crucial building block in synthesizing quinoxaline derivatives with potential antitumor activity [, ]. Its structure allows for the introduction of various substituents, facilitating the exploration of structure-activity relationships and the development of more potent and selective antitumor agents.
Q2: Can you elaborate on the synthesis of quinoxaline derivatives using this compound, as highlighted in the research papers?
A: Both studies utilize this compound as a key intermediate. In one study [], it's reacted with 2,4-pyridinediol to introduce a pyridine ring into the quinoxaline structure. The other study [] explores a broader approach, reacting this compound with various phenolic compounds to generate a series of diverse quinoxaline derivatives. This strategy allows for the systematic evaluation of how different substituents affect the antitumor activity of the resulting compounds.
Q3: What analytical techniques were employed to characterize the synthesized quinoxaline derivatives?
A: Both research papers employed a combination of analytical techniques to confirm the structures of the synthesized compounds. These techniques included elemental analysis, ¹H Nuclear Magnetic Resonance (¹H NMR) spectroscopy, Mass Spectrometry (MS), and Infrared (IR) spectroscopy [, ]. These methods provide complementary information about the elemental composition, proton environment, molecular weight, and functional groups present in the synthesized molecules, respectively.
Disclaimer and Information on In-Vitro Research Products
Please be aware that all articles and product information presented on BenchChem are intended solely for informational purposes. The products available for purchase on BenchChem are specifically designed for in-vitro studies, which are conducted outside of living organisms. In-vitro studies, derived from the Latin term "in glass," involve experiments performed in controlled laboratory settings using cells or tissues. It is important to note that these products are not categorized as medicines or drugs, and they have not received approval from the FDA for the prevention, treatment, or cure of any medical condition, ailment, or disease. We must emphasize that any form of bodily introduction of these products into humans or animals is strictly prohibited by law. It is essential to adhere to these guidelines to ensure compliance with legal and ethical standards in research and experimentation.