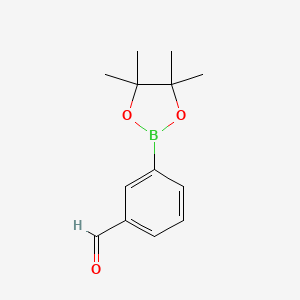
3-(4,4,5,5-Tetramethyl-1,3,2-dioxaborolan-2-yl)benzaldehyde
Overview
Description
3-(4,4,5,5-Tetramethyl-1,3,2-dioxaborolan-2-yl)benzaldehyde: is an organic compound that features a benzaldehyde moiety attached to a dioxaborolane ring. This compound is notable for its utility in organic synthesis, particularly in the formation of carbon-carbon bonds through cross-coupling reactions. It is often used as a building block in the synthesis of more complex molecules.
Mechanism of Action
Target of Action
Similar compounds, such as 4,4,5,5-tetramethyl-1,3,2-dioxaborolane, are known to interact with alkylbenzenes and alkyl or aryl alkynes and alkenes .
Mode of Action
Compounds with similar structures, like 4,4,5,5-tetramethyl-1,3,2-dioxaborolane, are known to participate in borylation reactions at the benzylic c-h bond of alkylbenzenes in the presence of a palladium catalyst to form pinacol benzyl boronate . They can also participate in hydroboration of alkyl or aryl alkynes and alkenes in the presence of transition metal catalysts .
Biochemical Pathways
The borylation and hydroboration reactions it participates in can affect various biochemical pathways, particularly those involving alkylbenzenes and alkyl or aryl alkynes and alkenes .
Result of Action
Its participation in borylation and hydroboration reactions suggests that it can contribute to the formation of complex organic compounds .
Action Environment
Similar compounds are known to be stable under inert gas and should be stored in a cool, dry place .
Preparation Methods
Synthetic Routes and Reaction Conditions: The synthesis of 3-(4,4,5,5-Tetramethyl-1,3,2-dioxaborolan-2-yl)benzaldehyde typically involves the borylation of benzaldehyde derivatives. One common method is the reaction of 4-bromobenzaldehyde with bis(pinacolato)diboron in the presence of a palladium catalyst and a base such as potassium acetate. The reaction is usually carried out in a solvent like dimethylformamide at elevated temperatures.
Industrial Production Methods: On an industrial scale, the production of this compound follows similar synthetic routes but is optimized for larger batch sizes. The use of continuous flow reactors and automated systems can enhance the efficiency and yield of the process. The reaction conditions are carefully controlled to ensure high purity and consistency of the final product.
Chemical Reactions Analysis
Types of Reactions:
Oxidation: The aldehyde group in 3-(4,4,5,5-Tetramethyl-1,3,2-dioxaborolan-2-yl)benzaldehyde can undergo oxidation to form the corresponding carboxylic acid.
Reduction: The aldehyde group can be reduced to the corresponding alcohol using reducing agents such as sodium borohydride or lithium aluminum hydride.
Substitution: The boronate ester group can participate in Suzuki-Miyaura cross-coupling reactions to form carbon-carbon bonds.
Common Reagents and Conditions:
Oxidation: Potassium permanganate or chromium trioxide in acidic conditions.
Reduction: Sodium borohydride in methanol or lithium aluminum hydride in ether.
Substitution: Palladium catalysts, such as palladium(II) acetate, in the presence of a base like potassium carbonate.
Major Products Formed:
Oxidation: 3-(4,4,5,5-Tetramethyl-1,3,2-dioxaborolan-2-yl)benzoic acid.
Reduction: 3-(4,4,5,5-Tetramethyl-1,3,2-dioxaborolan-2-yl)benzyl alcohol.
Substitution: Various biaryl compounds depending on the coupling partner used.
Scientific Research Applications
Chemistry: 3-(4,4,5,5-Tetramethyl-1,3,2-dioxaborolan-2-yl)benzaldehyde is widely used in organic synthesis, particularly in the formation of biaryl compounds through Suzuki-Miyaura cross-coupling reactions. It serves as a versatile building block for the synthesis of pharmaceuticals, agrochemicals, and advanced materials.
Biology and Medicine: In medicinal chemistry, this compound is used to synthesize biologically active molecules. Its derivatives have been investigated for their potential as therapeutic agents in various diseases, including cancer and infectious diseases.
Industry: In the industrial sector, this compound is used in the production of polymers and advanced materials. Its ability to form stable carbon-carbon bonds makes it valuable in the synthesis of complex organic molecules.
Comparison with Similar Compounds
- 4-(4,4,5,5-Tetramethyl-1,3,2-dioxaborolan-2-yl)benzene
- 4-(4,4,5,5-Tetramethyl-1,3,2-dioxaborolan-2-yl)benzoic acid
- 4-(4,4,5,5-Tetramethyl-1,3,2-dioxaborolan-2-yl)benzyl alcohol
Uniqueness: 3-(4,4,5,5-Tetramethyl-1,3,2-dioxaborolan-2-yl)benzaldehyde is unique due to the presence of both an aldehyde group and a boronate ester group. This dual functionality allows it to participate in a wider range of chemical reactions compared to its analogs, making it a highly versatile compound in organic synthesis.
Properties
IUPAC Name |
3-(4,4,5,5-tetramethyl-1,3,2-dioxaborolan-2-yl)benzaldehyde | |
---|---|---|
Source | PubChem | |
URL | https://pubchem.ncbi.nlm.nih.gov | |
Description | Data deposited in or computed by PubChem | |
InChI |
InChI=1S/C13H17BO3/c1-12(2)13(3,4)17-14(16-12)11-7-5-6-10(8-11)9-15/h5-9H,1-4H3 | |
Source | PubChem | |
URL | https://pubchem.ncbi.nlm.nih.gov | |
Description | Data deposited in or computed by PubChem | |
InChI Key |
IFYMOLFMYIDYEN-UHFFFAOYSA-N | |
Source | PubChem | |
URL | https://pubchem.ncbi.nlm.nih.gov | |
Description | Data deposited in or computed by PubChem | |
Canonical SMILES |
B1(OC(C(O1)(C)C)(C)C)C2=CC(=CC=C2)C=O | |
Source | PubChem | |
URL | https://pubchem.ncbi.nlm.nih.gov | |
Description | Data deposited in or computed by PubChem | |
Molecular Formula |
C13H17BO3 | |
Source | PubChem | |
URL | https://pubchem.ncbi.nlm.nih.gov | |
Description | Data deposited in or computed by PubChem | |
DSSTOX Substance ID |
DTXSID00375240 | |
Record name | 3-(4,4,5,5-Tetramethyl-1,3,2-dioxaborolan-2-yl)benzaldehyde | |
Source | EPA DSSTox | |
URL | https://comptox.epa.gov/dashboard/DTXSID00375240 | |
Description | DSSTox provides a high quality public chemistry resource for supporting improved predictive toxicology. | |
Molecular Weight |
232.09 g/mol | |
Source | PubChem | |
URL | https://pubchem.ncbi.nlm.nih.gov | |
Description | Data deposited in or computed by PubChem | |
CAS No. |
380151-86-0 | |
Record name | 3-(4,4,5,5-Tetramethyl-1,3,2-dioxaborolan-2-yl)benzaldehyde | |
Source | EPA DSSTox | |
URL | https://comptox.epa.gov/dashboard/DTXSID00375240 | |
Description | DSSTox provides a high quality public chemistry resource for supporting improved predictive toxicology. | |
Record name | 3-Formylphenylboronic acid pinacol ester | |
Source | European Chemicals Agency (ECHA) | |
URL | https://echa.europa.eu/information-on-chemicals | |
Description | The European Chemicals Agency (ECHA) is an agency of the European Union which is the driving force among regulatory authorities in implementing the EU's groundbreaking chemicals legislation for the benefit of human health and the environment as well as for innovation and competitiveness. | |
Explanation | Use of the information, documents and data from the ECHA website is subject to the terms and conditions of this Legal Notice, and subject to other binding limitations provided for under applicable law, the information, documents and data made available on the ECHA website may be reproduced, distributed and/or used, totally or in part, for non-commercial purposes provided that ECHA is acknowledged as the source: "Source: European Chemicals Agency, http://echa.europa.eu/". Such acknowledgement must be included in each copy of the material. ECHA permits and encourages organisations and individuals to create links to the ECHA website under the following cumulative conditions: Links can only be made to webpages that provide a link to the Legal Notice page. | |
Retrosynthesis Analysis
AI-Powered Synthesis Planning: Our tool employs the Template_relevance Pistachio, Template_relevance Bkms_metabolic, Template_relevance Pistachio_ringbreaker, Template_relevance Reaxys, Template_relevance Reaxys_biocatalysis model, leveraging a vast database of chemical reactions to predict feasible synthetic routes.
One-Step Synthesis Focus: Specifically designed for one-step synthesis, it provides concise and direct routes for your target compounds, streamlining the synthesis process.
Accurate Predictions: Utilizing the extensive PISTACHIO, BKMS_METABOLIC, PISTACHIO_RINGBREAKER, REAXYS, REAXYS_BIOCATALYSIS database, our tool offers high-accuracy predictions, reflecting the latest in chemical research and data.
Strategy Settings
Precursor scoring | Relevance Heuristic |
---|---|
Min. plausibility | 0.01 |
Model | Template_relevance |
Template Set | Pistachio/Bkms_metabolic/Pistachio_ringbreaker/Reaxys/Reaxys_biocatalysis |
Top-N result to add to graph | 6 |
Feasible Synthetic Routes
Disclaimer and Information on In-Vitro Research Products
Please be aware that all articles and product information presented on BenchChem are intended solely for informational purposes. The products available for purchase on BenchChem are specifically designed for in-vitro studies, which are conducted outside of living organisms. In-vitro studies, derived from the Latin term "in glass," involve experiments performed in controlled laboratory settings using cells or tissues. It is important to note that these products are not categorized as medicines or drugs, and they have not received approval from the FDA for the prevention, treatment, or cure of any medical condition, ailment, or disease. We must emphasize that any form of bodily introduction of these products into humans or animals is strictly prohibited by law. It is essential to adhere to these guidelines to ensure compliance with legal and ethical standards in research and experimentation.