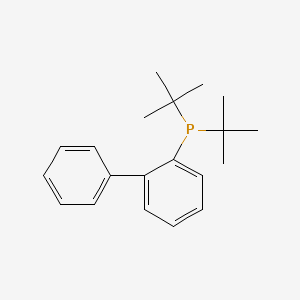
2-(Di-tert-butylphosphino)biphenyl
Overview
Description
2-(Di-tert-butylphosphino)biphenyl, also known as (2-Biphenyl)di-tert-butylphosphine, is a sterically bulky biaryl phosphine ligand. It is a reactive dialkylbiaryl phosphine ligand that catalyzes carbon-nitrogen bond-forming reactions. This compound is widely used in various catalytic processes due to its unique structural features and reactivity .
Mechanism of Action
Target of Action
2-(Di-tert-butylphosphino)biphenyl, also known as JohnPhos, is an organophosphine ligand . Its primary targets are transition metals, with which it forms coordination complexes .
Mode of Action
JohnPhos interacts with its targets (transition metals) through coordination, forming a complex . This interaction facilitates various catalytic reactions, including carbon-nitrogen bond-forming reactions .
Biochemical Pathways
The biochemical pathways affected by JohnPhos are primarily those involving carbon-nitrogen bond formation . The compound acts as a catalyst in these reactions, accelerating the rate at which they occur .
Result of Action
The molecular and cellular effects of JohnPhos’s action primarily involve the formation of carbon-nitrogen bonds . This can lead to the synthesis of various organic compounds, depending on the specific reaction conditions and reactants present .
Biochemical Analysis
Biochemical Properties
JohnPhos plays a crucial role in biochemical reactions as a ligand. It interacts with various enzymes and proteins, particularly those involved in catalytic processes. For instance, JohnPhos is known to interact with palladium catalysts, enhancing their reactivity and stability. The nature of these interactions involves the coordination of the phosphine ligand to the metal center, which facilitates the formation of carbon-nitrogen bonds in reactions such as the Buchwald-Hartwig cross-coupling .
Cellular Effects
JohnPhos influences various cellular processes, particularly those related to cell signaling and metabolism. In cellular environments, JohnPhos can affect the function of enzymes involved in catalytic reactions, thereby influencing cellular metabolism. Additionally, JohnPhos may impact gene expression by modulating the activity of transcription factors and other regulatory proteins. These effects can lead to changes in cellular function and behavior .
Molecular Mechanism
The molecular mechanism of JohnPhos involves its binding interactions with biomolecules, particularly metal catalysts. JohnPhos acts as a ligand, coordinating to the metal center and stabilizing the catalytic complex. This coordination enhances the reactivity of the metal catalyst, facilitating various chemical transformations. Additionally, JohnPhos can influence enzyme activity by either inhibiting or activating specific catalytic sites, leading to changes in gene expression and cellular function .
Temporal Effects in Laboratory Settings
In laboratory settings, the effects of JohnPhos can change over time due to its stability and degradation. JohnPhos is generally stable under standard laboratory conditions, but its reactivity may decrease over extended periods. Long-term studies have shown that JohnPhos can maintain its catalytic activity for several hours, but prolonged exposure to harsh conditions may lead to degradation and reduced efficacy. These temporal effects are important considerations for in vitro and in vivo studies .
Dosage Effects in Animal Models
The effects of JohnPhos vary with different dosages in animal models. At low doses, JohnPhos can effectively facilitate catalytic reactions without causing significant adverse effects. At high doses, JohnPhos may exhibit toxic effects, including cellular toxicity and disruption of normal cellular processes. Threshold effects have been observed, where a specific dosage range is required to achieve optimal catalytic activity without causing harm to the organism .
Metabolic Pathways
JohnPhos is involved in various metabolic pathways, particularly those related to catalytic reactions. It interacts with enzymes and cofactors involved in these pathways, influencing metabolic flux and metabolite levels. For example, JohnPhos can enhance the activity of palladium catalysts in the Suzuki-Miyaura coupling reaction, leading to increased production of desired metabolites. These interactions are crucial for understanding the role of JohnPhos in metabolic processes .
Transport and Distribution
Within cells and tissues, JohnPhos is transported and distributed through interactions with transporters and binding proteins. These interactions facilitate the localization and accumulation of JohnPhos in specific cellular compartments. The distribution of JohnPhos can influence its activity and function, as it needs to be localized to the appropriate sites to exert its catalytic effects. Understanding the transport and distribution of JohnPhos is essential for optimizing its use in biochemical applications .
Subcellular Localization
JohnPhos exhibits specific subcellular localization, which can affect its activity and function. Targeting signals and post-translational modifications may direct JohnPhos to particular compartments or organelles within the cell. For instance, JohnPhos may be localized to the cytoplasm or nucleus, where it can interact with enzymes and other biomolecules involved in catalytic reactions. The subcellular localization of JohnPhos is a key factor in determining its efficacy and role in biochemical processes .
Preparation Methods
2-(Di-tert-butylphosphino)biphenyl can be synthesized through several synthetic routes. One common method involves the reaction of biphenyl with di-tert-butylphosphine under specific conditions. The reaction typically requires a palladium catalyst and is carried out under an inert atmosphere to prevent oxidation . Industrial production methods for this compound involve similar synthetic routes but are optimized for large-scale production, ensuring high yield and purity.
Chemical Reactions Analysis
2-(Di-tert-butylphosphino)biphenyl undergoes various types of chemical reactions, including:
Cross Couplings: This compound is used as a ligand in palladium-catalyzed cross-coupling reactions such as Suzuki-Miyaura, Heck, and Buchwald-Hartwig reactions
Oxidation and Reduction: While this compound itself is not typically involved in oxidation or reduction reactions, it can facilitate these processes when used as a ligand in catalytic systems.
Substitution: This compound can participate in substitution reactions, particularly in the formation of carbon-nitrogen bonds.
Common reagents and conditions used in these reactions include palladium catalysts, aryl halides, and bases such as potassium carbonate. Major products formed from these reactions are often complex organic molecules with applications in pharmaceuticals and materials science .
Scientific Research Applications
2-(Di-tert-butylphosphino)biphenyl has a wide range of scientific research applications:
Chemistry: this compound is extensively used in organic synthesis, particularly in the formation of carbon-nitrogen bonds. .
Industry: This compound is used in the production of fine chemicals, agrochemicals, and materials science.
Comparison with Similar Compounds
2-(Di-tert-butylphosphino)biphenyl is part of the Buchwald ligand family, which includes several other sterically bulky biaryl phosphine ligands. Similar compounds include:
XPhos: Another biaryl phosphine ligand with similar applications in cross-coupling reactions.
SPhos: Known for its use in palladium-catalyzed amination reactions.
tBuXPhos: A ligand with tert-butyl groups that provides steric bulk and electron-donating properties.
Cythis compound: A cyclohexyl-substituted version of this compound with enhanced stability.
This compound is unique due to its specific steric and electronic properties, which make it particularly effective in certain catalytic processes. Its ability to stabilize reactive intermediates and facilitate challenging transformations sets it apart from other ligands in the Buchwald family .
Properties
IUPAC Name |
ditert-butyl-(2-phenylphenyl)phosphane | |
---|---|---|
Source | PubChem | |
URL | https://pubchem.ncbi.nlm.nih.gov | |
Description | Data deposited in or computed by PubChem | |
InChI |
InChI=1S/C20H27P/c1-19(2,3)21(20(4,5)6)18-15-11-10-14-17(18)16-12-8-7-9-13-16/h7-15H,1-6H3 | |
Source | PubChem | |
URL | https://pubchem.ncbi.nlm.nih.gov | |
Description | Data deposited in or computed by PubChem | |
InChI Key |
CNXMDTWQWLGCPE-UHFFFAOYSA-N | |
Source | PubChem | |
URL | https://pubchem.ncbi.nlm.nih.gov | |
Description | Data deposited in or computed by PubChem | |
Canonical SMILES |
CC(C)(C)P(C1=CC=CC=C1C2=CC=CC=C2)C(C)(C)C | |
Source | PubChem | |
URL | https://pubchem.ncbi.nlm.nih.gov | |
Description | Data deposited in or computed by PubChem | |
Molecular Formula |
C20H27P | |
Source | PubChem | |
URL | https://pubchem.ncbi.nlm.nih.gov | |
Description | Data deposited in or computed by PubChem | |
DSSTOX Substance ID |
DTXSID50370169 | |
Record name | 2-(Di-tert-butylphosphino)biphenyl | |
Source | EPA DSSTox | |
URL | https://comptox.epa.gov/dashboard/DTXSID50370169 | |
Description | DSSTox provides a high quality public chemistry resource for supporting improved predictive toxicology. | |
Molecular Weight |
298.4 g/mol | |
Source | PubChem | |
URL | https://pubchem.ncbi.nlm.nih.gov | |
Description | Data deposited in or computed by PubChem | |
CAS No. |
224311-51-7 | |
Record name | 2-(Di-tert-butylphosphino)biphenyl | |
Source | CAS Common Chemistry | |
URL | https://commonchemistry.cas.org/detail?cas_rn=224311-51-7 | |
Description | CAS Common Chemistry is an open community resource for accessing chemical information. Nearly 500,000 chemical substances from CAS REGISTRY cover areas of community interest, including common and frequently regulated chemicals, and those relevant to high school and undergraduate chemistry classes. This chemical information, curated by our expert scientists, is provided in alignment with our mission as a division of the American Chemical Society. | |
Explanation | The data from CAS Common Chemistry is provided under a CC-BY-NC 4.0 license, unless otherwise stated. | |
Record name | 2-(Di-tert-butylphosphino)biphenyl | |
Source | ChemIDplus | |
URL | https://pubchem.ncbi.nlm.nih.gov/substance/?source=chemidplus&sourceid=0224311517 | |
Description | ChemIDplus is a free, web search system that provides access to the structure and nomenclature authority files used for the identification of chemical substances cited in National Library of Medicine (NLM) databases, including the TOXNET system. | |
Record name | 2-(Di-tert-butylphosphino)biphenyl | |
Source | EPA DSSTox | |
URL | https://comptox.epa.gov/dashboard/DTXSID50370169 | |
Description | DSSTox provides a high quality public chemistry resource for supporting improved predictive toxicology. | |
Record name | 2-(DI-TERT-BUTYLPHOSPHINO)BIPHENYL | |
Source | FDA Global Substance Registration System (GSRS) | |
URL | https://gsrs.ncats.nih.gov/ginas/app/beta/substances/5Y743P380H | |
Description | The FDA Global Substance Registration System (GSRS) enables the efficient and accurate exchange of information on what substances are in regulated products. Instead of relying on names, which vary across regulatory domains, countries, and regions, the GSRS knowledge base makes it possible for substances to be defined by standardized, scientific descriptions. | |
Explanation | Unless otherwise noted, the contents of the FDA website (www.fda.gov), both text and graphics, are not copyrighted. They are in the public domain and may be republished, reprinted and otherwise used freely by anyone without the need to obtain permission from FDA. Credit to the U.S. Food and Drug Administration as the source is appreciated but not required. | |
Synthesis routes and methods I
Procedure details
Synthesis routes and methods II
Procedure details
Retrosynthesis Analysis
AI-Powered Synthesis Planning: Our tool employs the Template_relevance Pistachio, Template_relevance Bkms_metabolic, Template_relevance Pistachio_ringbreaker, Template_relevance Reaxys, Template_relevance Reaxys_biocatalysis model, leveraging a vast database of chemical reactions to predict feasible synthetic routes.
One-Step Synthesis Focus: Specifically designed for one-step synthesis, it provides concise and direct routes for your target compounds, streamlining the synthesis process.
Accurate Predictions: Utilizing the extensive PISTACHIO, BKMS_METABOLIC, PISTACHIO_RINGBREAKER, REAXYS, REAXYS_BIOCATALYSIS database, our tool offers high-accuracy predictions, reflecting the latest in chemical research and data.
Strategy Settings
Precursor scoring | Relevance Heuristic |
---|---|
Min. plausibility | 0.01 |
Model | Template_relevance |
Template Set | Pistachio/Bkms_metabolic/Pistachio_ringbreaker/Reaxys/Reaxys_biocatalysis |
Top-N result to add to graph | 6 |
Feasible Synthetic Routes
Disclaimer and Information on In-Vitro Research Products
Please be aware that all articles and product information presented on BenchChem are intended solely for informational purposes. The products available for purchase on BenchChem are specifically designed for in-vitro studies, which are conducted outside of living organisms. In-vitro studies, derived from the Latin term "in glass," involve experiments performed in controlled laboratory settings using cells or tissues. It is important to note that these products are not categorized as medicines or drugs, and they have not received approval from the FDA for the prevention, treatment, or cure of any medical condition, ailment, or disease. We must emphasize that any form of bodily introduction of these products into humans or animals is strictly prohibited by law. It is essential to adhere to these guidelines to ensure compliance with legal and ethical standards in research and experimentation.