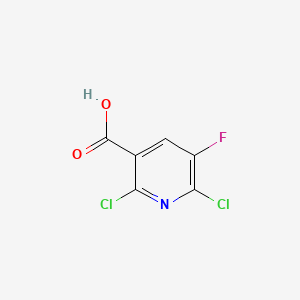
2,6-Dichloro-5-fluoronicotinic acid
Overview
Description
2,6-Dichloro-5-fluoronicotinic Acid, also known as 2,6-DCFNA, is a halogenated heterocyclic compound . It is a chlorinating agent that is activated in acidic solutions . It is used to produce the disinfectant peracetic acid and in the industrial process of producing phenol .
Synthesis Analysis
The synthesis of 2,6-Dichloro-5-fluoronicotinic acid has been reported from ethyl 2,6-dichloro-5-fluoropyridine-3-carboxylate . An improved process for the synthesis of 2,6-Dichloro-5-fluoronicotinic acid has also been documented .Molecular Structure Analysis
The molecular formula of 2,6-Dichloro-5-fluoronicotinic Acid is C6H2Cl2FNO2 . The IUPAC name is 2,6-dichloro-5-fluoropyridine-3-carboxylic acid .Chemical Reactions Analysis
2,6-Dichloro-5-fluoronicotinic acid (2,6-DCFNA) is a chlorinating agent that is activated in acidic solutions . It reacts with chloride in the production of disinfectant peracetic acid and in the industrial process of producing phenol .Physical And Chemical Properties Analysis
2,6-Dichloro-5-fluoronicotinic Acid has a molecular weight of 209.99 g/mol . It has a density of 1.6207 g/cm3 at 25°C . The boiling point is 335.3±37.0 °C at 760 mmHg . The vapor pressure is 0.0±0.8 mmHg at 25°C .Scientific Research Applications
Synthesis of Third-Generation Quinolones
2,6-Dichloro-5-fluoronicotinic acid is an important intermediate in the synthesis of third-generation quinolone drugs . These drugs are a type of antibiotics that are effective against a broad range of bacteria and are often used when other antibiotics fail.
Preparation of Fluorinated Naphthyridine Derivatives
This compound is used as an intermediate in the preparation of fluorinated naphthyridine derivatives . These derivatives have potential applications in the development of new antimicrobial agents.
Modulation of Protein-Protein Interactions
2,6-Dichloro-5-fluoronicotinic acid can be used as a reagent for the modulation of protein-protein interactions . This can help researchers understand the mechanisms involved in amyloid assembly, which is relevant to diseases like Alzheimer’s.
4. Preparation of Self-Assembled Monolayer (SAM) Compounds This compound may be used in the preparation of self-assembled monolayer (SAM) compounds . SAMs have applications in various fields, including biosensors, microelectronics, and nanotechnology.
Life Science Research
2,6-Dichloro-5-fluoronicotinic acid is a biochemical reagent that can be used as a biological material or organic compound for life science related research .
Synthesis of Tosufloxacin Tosilate Impurity
This compound is also used in the synthesis of Tosufloxacin tosilate impurity . Tosufloxacin tosilate is a type of antibiotic, and understanding its impurities is important for quality control in pharmaceutical manufacturing.
Mechanism of Action
Target of Action
It is suggested that the compound may modulate protein-protein interactions .
Mode of Action
It is suggested that it may interact with its targets to modulate protein-protein interactions
Biochemical Pathways
Given its potential role in modulating protein-protein interactions , it may influence various biochemical pathways depending on the specific proteins it interacts with.
Result of Action
Given its potential role in modulating protein-protein interactions , it may have diverse effects depending on the specific proteins and cells involved.
Safety and Hazards
2,6-Dichloro-5-fluoronicotinic Acid is considered hazardous. It causes skin irritation and serious eye irritation. It may also cause respiratory irritation . Safety measures include avoiding dust formation, avoiding breathing mist, gas or vapors, avoiding contact with skin and eye, using personal protective equipment, wearing chemical impermeable gloves, and ensuring adequate ventilation .
properties
IUPAC Name |
2,6-dichloro-5-fluoropyridine-3-carboxylic acid | |
---|---|---|
Source | PubChem | |
URL | https://pubchem.ncbi.nlm.nih.gov | |
Description | Data deposited in or computed by PubChem | |
InChI |
InChI=1S/C6H2Cl2FNO2/c7-4-2(6(11)12)1-3(9)5(8)10-4/h1H,(H,11,12) | |
Source | PubChem | |
URL | https://pubchem.ncbi.nlm.nih.gov | |
Description | Data deposited in or computed by PubChem | |
InChI Key |
LTDGKGCHRNNCAC-UHFFFAOYSA-N | |
Source | PubChem | |
URL | https://pubchem.ncbi.nlm.nih.gov | |
Description | Data deposited in or computed by PubChem | |
Canonical SMILES |
C1=C(C(=NC(=C1F)Cl)Cl)C(=O)O | |
Source | PubChem | |
URL | https://pubchem.ncbi.nlm.nih.gov | |
Description | Data deposited in or computed by PubChem | |
Molecular Formula |
C6H2Cl2FNO2 | |
Source | PubChem | |
URL | https://pubchem.ncbi.nlm.nih.gov | |
Description | Data deposited in or computed by PubChem | |
DSSTOX Substance ID |
DTXSID00369962 | |
Record name | 2,6-Dichloro-5-fluoronicotinic acid | |
Source | EPA DSSTox | |
URL | https://comptox.epa.gov/dashboard/DTXSID00369962 | |
Description | DSSTox provides a high quality public chemistry resource for supporting improved predictive toxicology. | |
Molecular Weight |
209.99 g/mol | |
Source | PubChem | |
URL | https://pubchem.ncbi.nlm.nih.gov | |
Description | Data deposited in or computed by PubChem | |
Product Name |
2,6-Dichloro-5-fluoronicotinic acid | |
CAS RN |
82671-06-5 | |
Record name | 2,6-Dichloro-5-fluoronicotinic acid | |
Source | CAS Common Chemistry | |
URL | https://commonchemistry.cas.org/detail?cas_rn=82671-06-5 | |
Description | CAS Common Chemistry is an open community resource for accessing chemical information. Nearly 500,000 chemical substances from CAS REGISTRY cover areas of community interest, including common and frequently regulated chemicals, and those relevant to high school and undergraduate chemistry classes. This chemical information, curated by our expert scientists, is provided in alignment with our mission as a division of the American Chemical Society. | |
Explanation | The data from CAS Common Chemistry is provided under a CC-BY-NC 4.0 license, unless otherwise stated. | |
Record name | 2,6-Dichloro-5-fluoronicotinic acid | |
Source | EPA DSSTox | |
URL | https://comptox.epa.gov/dashboard/DTXSID00369962 | |
Description | DSSTox provides a high quality public chemistry resource for supporting improved predictive toxicology. | |
Record name | 2,6-Dichloro-5-fluoronicotinic acid | |
Source | European Chemicals Agency (ECHA) | |
URL | https://echa.europa.eu/information-on-chemicals | |
Description | The European Chemicals Agency (ECHA) is an agency of the European Union which is the driving force among regulatory authorities in implementing the EU's groundbreaking chemicals legislation for the benefit of human health and the environment as well as for innovation and competitiveness. | |
Explanation | Use of the information, documents and data from the ECHA website is subject to the terms and conditions of this Legal Notice, and subject to other binding limitations provided for under applicable law, the information, documents and data made available on the ECHA website may be reproduced, distributed and/or used, totally or in part, for non-commercial purposes provided that ECHA is acknowledged as the source: "Source: European Chemicals Agency, http://echa.europa.eu/". Such acknowledgement must be included in each copy of the material. ECHA permits and encourages organisations and individuals to create links to the ECHA website under the following cumulative conditions: Links can only be made to webpages that provide a link to the Legal Notice page. | |
Synthesis routes and methods I
Procedure details
Synthesis routes and methods II
Procedure details
Synthesis routes and methods III
Procedure details
Synthesis routes and methods IV
Procedure details
Synthesis routes and methods V
Procedure details
Retrosynthesis Analysis
AI-Powered Synthesis Planning: Our tool employs the Template_relevance Pistachio, Template_relevance Bkms_metabolic, Template_relevance Pistachio_ringbreaker, Template_relevance Reaxys, Template_relevance Reaxys_biocatalysis model, leveraging a vast database of chemical reactions to predict feasible synthetic routes.
One-Step Synthesis Focus: Specifically designed for one-step synthesis, it provides concise and direct routes for your target compounds, streamlining the synthesis process.
Accurate Predictions: Utilizing the extensive PISTACHIO, BKMS_METABOLIC, PISTACHIO_RINGBREAKER, REAXYS, REAXYS_BIOCATALYSIS database, our tool offers high-accuracy predictions, reflecting the latest in chemical research and data.
Strategy Settings
Precursor scoring | Relevance Heuristic |
---|---|
Min. plausibility | 0.01 |
Model | Template_relevance |
Template Set | Pistachio/Bkms_metabolic/Pistachio_ringbreaker/Reaxys/Reaxys_biocatalysis |
Top-N result to add to graph | 6 |
Feasible Synthetic Routes
Q & A
Q1: What are the main applications of 2,6-Dichloro-5-fluoronicotinic acid in pharmaceutical synthesis?
A: 2,6-Dichloro-5-fluoronicotinic acid serves as a versatile precursor in synthesizing various pharmaceutical compounds. One notable example is its use in the production of Enoxacin, a potent antibacterial agent. [] This synthesis involves a multi-step process, including Dieckmann-type cyclization, to transform derivatives of 2,6-Dichloro-5-fluoronicotinic acid into the desired 1,8-naphthyridine structure of Enoxacin. []
Q2: Can you describe a specific synthetic route involving 2,6-Dichloro-5-fluoronicotinic acid?
A: One example is the synthesis of ethyl 2,6-dichloro-5-fluoronicotinoyl acetate. [] This process begins by converting 2,6-Dichloro-5-fluoronicotinic acid to its corresponding acid chloride using thionyl chloride. [] Subsequently, this acid chloride reacts with potassium ethyl malonate in the presence of magnesium chloride and triethylamine to yield the desired ethyl 2,6-dichloro-5-fluoronicotinoyl acetate. [] The overall yield for this two-step synthesis is approximately 71%. []
Q3: How is 2,6-Dichloro-5-fluoronicotinic acid utilized in synthesizing complex heterocyclic compounds?
A: A notable application is its role in synthesizing 2-[(1H-pyrrolo[2,3-b]pyridine-4-yl)methylamino]-5-fluoronicotinic acid (compound 1), a key pharmaceutical intermediate. [] Researchers achieved this by first converting 2,6-Dichloro-5-fluoronicotinic acid to 2-chloro-5-fluoronicotinic acid (compound 3) through a highly selective monodechlorination reaction. [] This compound then reacts with a specifically prepared 7-azaindole derivative, containing an aminomethyl moiety, to yield the final product, compound 1. [] This synthesis highlights the importance of 2,6-Dichloro-5-fluoronicotinic acid's unique structure in facilitating the preparation of complex pharmaceutical intermediates. []
Disclaimer and Information on In-Vitro Research Products
Please be aware that all articles and product information presented on BenchChem are intended solely for informational purposes. The products available for purchase on BenchChem are specifically designed for in-vitro studies, which are conducted outside of living organisms. In-vitro studies, derived from the Latin term "in glass," involve experiments performed in controlled laboratory settings using cells or tissues. It is important to note that these products are not categorized as medicines or drugs, and they have not received approval from the FDA for the prevention, treatment, or cure of any medical condition, ailment, or disease. We must emphasize that any form of bodily introduction of these products into humans or animals is strictly prohibited by law. It is essential to adhere to these guidelines to ensure compliance with legal and ethical standards in research and experimentation.