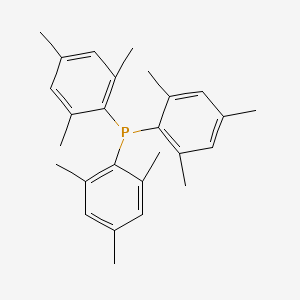
Trimesitylphosphine
Overview
Description
Preparation Methods
Trimesitylphosphine is typically synthesized through the reaction of triisopropylidenebromobenzene with sodium phosphide at low temperatures . The compound can be purified by recrystallization from ethanol . Industrial production methods often involve similar synthetic routes, ensuring high purity and yield.
Chemical Reactions Analysis
Trimesitylphosphine undergoes various chemical reactions, including:
Oxidation: It can be oxidized to this compound oxide using reagents like hydrogen peroxide.
Substitution: It participates in substitution reactions, forming stable complexes with transition metals.
Coupling Reactions: It is a suitable ligand for Buchwald-Hartwig cross-coupling, Suzuki-Miyaura coupling, Stille coupling, Sonogashira coupling, Negishi coupling, Heck coupling, and Hiyama coupling reactions.
Common reagents and conditions for these reactions include organic solvents, transition metal catalysts, and controlled temperatures. The major products formed depend on the specific reaction and reagents used.
Scientific Research Applications
Trimesitylphosphine has a wide range of applications in scientific research:
Mechanism of Action
The mechanism of action of trimesitylphosphine involves its ability to form stable complexes with transition metals, which then participate in catalytic reactions. These complexes can facilitate various chemical transformations by stabilizing reaction intermediates and lowering activation energies . The molecular targets and pathways involved depend on the specific application and reaction conditions.
Comparison with Similar Compounds
Trimesitylphosphine is unique due to its bulky structure and stability. Similar compounds include:
Triphenylphosphine: Commonly used in similar catalytic reactions but has a less bulky structure.
Tris(pentafluorophenyl)phosphine: Known for its electron-withdrawing properties, making it suitable for different types of reactions.
Tri(o-tolyl)phosphine: Another bulky phosphine ligand with applications in catalysis.
This compound stands out due to its specific steric and electronic properties, which make it particularly effective in forming stable metal complexes and facilitating various catalytic processes.
Properties
IUPAC Name |
tris(2,4,6-trimethylphenyl)phosphane | |
---|---|---|
Source | PubChem | |
URL | https://pubchem.ncbi.nlm.nih.gov | |
Description | Data deposited in or computed by PubChem | |
InChI |
InChI=1S/C27H33P/c1-16-10-19(4)25(20(5)11-16)28(26-21(6)12-17(2)13-22(26)7)27-23(8)14-18(3)15-24(27)9/h10-15H,1-9H3 | |
Source | PubChem | |
URL | https://pubchem.ncbi.nlm.nih.gov | |
Description | Data deposited in or computed by PubChem | |
InChI Key |
IDXDWPWXHTXJMZ-UHFFFAOYSA-N | |
Source | PubChem | |
URL | https://pubchem.ncbi.nlm.nih.gov | |
Description | Data deposited in or computed by PubChem | |
Canonical SMILES |
CC1=CC(=C(C(=C1)C)P(C2=C(C=C(C=C2C)C)C)C3=C(C=C(C=C3C)C)C)C | |
Source | PubChem | |
URL | https://pubchem.ncbi.nlm.nih.gov | |
Description | Data deposited in or computed by PubChem | |
Molecular Formula |
C27H33P | |
Source | PubChem | |
URL | https://pubchem.ncbi.nlm.nih.gov | |
Description | Data deposited in or computed by PubChem | |
DSSTOX Substance ID |
DTXSID00369902 | |
Record name | Trimesitylphosphine | |
Source | EPA DSSTox | |
URL | https://comptox.epa.gov/dashboard/DTXSID00369902 | |
Description | DSSTox provides a high quality public chemistry resource for supporting improved predictive toxicology. | |
Molecular Weight |
388.5 g/mol | |
Source | PubChem | |
URL | https://pubchem.ncbi.nlm.nih.gov | |
Description | Data deposited in or computed by PubChem | |
CAS No. |
23897-15-6 | |
Record name | Trimesitylphosphine | |
Source | EPA DSSTox | |
URL | https://comptox.epa.gov/dashboard/DTXSID00369902 | |
Description | DSSTox provides a high quality public chemistry resource for supporting improved predictive toxicology. | |
Retrosynthesis Analysis
AI-Powered Synthesis Planning: Our tool employs the Template_relevance Pistachio, Template_relevance Bkms_metabolic, Template_relevance Pistachio_ringbreaker, Template_relevance Reaxys, Template_relevance Reaxys_biocatalysis model, leveraging a vast database of chemical reactions to predict feasible synthetic routes.
One-Step Synthesis Focus: Specifically designed for one-step synthesis, it provides concise and direct routes for your target compounds, streamlining the synthesis process.
Accurate Predictions: Utilizing the extensive PISTACHIO, BKMS_METABOLIC, PISTACHIO_RINGBREAKER, REAXYS, REAXYS_BIOCATALYSIS database, our tool offers high-accuracy predictions, reflecting the latest in chemical research and data.
Strategy Settings
Precursor scoring | Relevance Heuristic |
---|---|
Min. plausibility | 0.01 |
Model | Template_relevance |
Template Set | Pistachio/Bkms_metabolic/Pistachio_ringbreaker/Reaxys/Reaxys_biocatalysis |
Top-N result to add to graph | 6 |
Feasible Synthetic Routes
Disclaimer and Information on In-Vitro Research Products
Please be aware that all articles and product information presented on BenchChem are intended solely for informational purposes. The products available for purchase on BenchChem are specifically designed for in-vitro studies, which are conducted outside of living organisms. In-vitro studies, derived from the Latin term "in glass," involve experiments performed in controlled laboratory settings using cells or tissues. It is important to note that these products are not categorized as medicines or drugs, and they have not received approval from the FDA for the prevention, treatment, or cure of any medical condition, ailment, or disease. We must emphasize that any form of bodily introduction of these products into humans or animals is strictly prohibited by law. It is essential to adhere to these guidelines to ensure compliance with legal and ethical standards in research and experimentation.