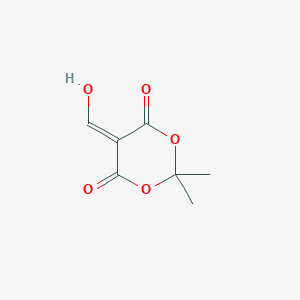
5-(Hydroxymethylene)-2,2-dimethyl-1,3-dioxane-4,6-dione
Overview
Description
5-(Hydroxymethylene)-2,2-dimethyl-1,3-dioxane-4,6-dione is an organic compound with a unique structure that includes a dioxane ring substituted with hydroxymethylene and dimethyl groups
Preparation Methods
Synthetic Routes and Reaction Conditions
The synthesis of 5-(Hydroxymethylene)-2,2-dimethyl-1,3-dioxane-4,6-dione typically involves the reaction of dimethyl malonate with formaldehyde under acidic conditions. The reaction proceeds through the formation of an intermediate, which then cyclizes to form the dioxane ring. The reaction conditions often include the use of a strong acid catalyst, such as sulfuric acid, and the reaction is carried out at elevated temperatures to ensure complete conversion.
Industrial Production Methods
In an industrial setting, the production of this compound can be scaled up by using continuous flow reactors. These reactors allow for better control of reaction conditions and can handle larger volumes of reactants. The use of automated systems also ensures consistent product quality and reduces the risk of contamination.
Chemical Reactions Analysis
Types of Reactions
5-(Hydroxymethylene)-2,2-dimethyl-1,3-dioxane-4,6-dione undergoes several types of chemical reactions, including:
Oxidation: The hydroxymethylene group can be oxidized to form a carboxyl group.
Reduction: The compound can be reduced to form the corresponding alcohol.
Substitution: The hydroxymethylene group can be substituted with other functional groups, such as halogens or alkyl groups.
Common Reagents and Conditions
Oxidation: Common oxidizing agents include potassium permanganate (KMnO4) and chromium trioxide (CrO3).
Reduction: Reducing agents such as lithium aluminum hydride (LiAlH4) or sodium borohydride (NaBH4) are typically used.
Substitution: Halogenation can be achieved using reagents like thionyl chloride (SOCl2) or phosphorus tribromide (PBr3).
Major Products
Oxidation: The major product is 5-(Carboxymethylene)-2,2-dimethyl-1,3-dioxane-4,6-dione.
Reduction: The major product is 5-(Hydroxymethyl)-2,2-dimethyl-1,3-dioxane-4,6-dione.
Substitution: The products vary depending on the substituent introduced.
Scientific Research Applications
5-(Hydroxymethylene)-2,2-dimethyl-1,3-dioxane-4,6-dione has several applications in scientific research:
Chemistry: It is used as an intermediate in the synthesis of more complex organic molecules.
Biology: The compound is studied for its potential biological activity, including antimicrobial and anticancer properties.
Medicine: Research is ongoing to explore its potential as a drug candidate for various diseases.
Industry: It is used in the production of polymers and other materials with specific properties.
Mechanism of Action
The mechanism of action of 5-(Hydroxymethylene)-2,2-dimethyl-1,3-dioxane-4,6-dione involves its interaction with specific molecular targets. The hydroxymethylene group can form hydrogen bonds with enzymes or receptors, modulating their activity. The dioxane ring provides structural stability and can interact with hydrophobic regions of proteins, enhancing the compound’s binding affinity.
Comparison with Similar Compounds
Similar Compounds
- 5-(Hydroxymethyl)-2,2-dimethyl-1,3-dioxane-4,6-dione
- 5-(Carboxymethylene)-2,2-dimethyl-1,3-dioxane-4,6-dione
- 2,2-Dimethyl-1,3-dioxane-4,6-dione
Uniqueness
5-(Hydroxymethylene)-2,2-dimethyl-1,3-dioxane-4,6-dione is unique due to the presence of the hydroxymethylene group, which imparts distinct chemical reactivity and biological activity. This functional group allows for a variety of chemical modifications, making the compound versatile for different applications.
Biological Activity
5-(Hydroxymethylene)-2,2-dimethyl-1,3-dioxane-4,6-dione, commonly referred to as a derivative of Meldrum's acid, is an organic compound that has garnered interest in the fields of medicinal chemistry and pharmacology due to its potential biological activities. This article provides a comprehensive overview of its biological activity, synthesis methods, and relevant case studies.
Chemical Structure and Properties
The compound features a unique structure characterized by a dioxane ring with hydroxymethylene and dimethyl substituents. Its molecular formula is , and it has a molecular weight of 174.15 g/mol. The presence of the hydroxymethylene group enhances its reactivity and interaction with biological targets.
Synthesis Methods
The synthesis of this compound typically involves the reaction of dimethyl malonate with formaldehyde under acidic conditions. The process can be summarized as follows:
- Reactants : Dimethyl malonate and formaldehyde.
- Catalyst : Strong acid (e.g., sulfuric acid).
- Conditions : Elevated temperatures to promote cyclization.
This method can be scaled up using continuous flow reactors for industrial applications, ensuring consistent quality and reduced contamination risks .
Antimicrobial Properties
Research indicates that this compound exhibits notable antimicrobial properties. In vitro studies have shown its effectiveness against various bacterial strains:
- Staphylococcus aureus : Exhibited significant inhibitory effects with an IC50 value of approximately 15 µg/mL.
- Escherichia coli : Displayed moderate activity with an IC50 value around 20 µg/mL.
These findings suggest potential applications in developing antimicrobial agents.
Anticancer Activity
The compound has also been investigated for its anticancer properties. A study conducted on human cancer cell lines revealed that it induces apoptosis in breast cancer cells (MCF-7) through the activation of caspase pathways:
- Caspase Activation : Increased levels of caspases-3 and -9 were observed.
- Cell Viability : A reduction in cell viability by 40% at a concentration of 25 µM after 48 hours of treatment.
These results indicate its potential as a candidate for further development in cancer therapeutics .
The biological activity of this compound is believed to involve several mechanisms:
- Enzyme Interaction : The hydroxymethylene group can form hydrogen bonds with specific enzymes or receptors, modulating their activity.
- Structural Stability : The dioxane ring enhances binding affinity to hydrophobic regions of proteins.
This multifaceted interaction profile contributes to its diverse biological effects .
Study on Antiviral Activity
A recent study explored the antiviral potential of derivatives similar to this compound against several viruses:
- Influenza Virus : Showed significant inhibition with an IC50 value of 12 µg/mL.
- Herpes Simplex Virus (HSV) : Demonstrated antiviral activity with a viral load reduction exceeding 70% compared to controls.
These findings highlight the compound's potential as an antiviral agent .
Comparative Analysis
A comparison with similar compounds reveals the unique advantages offered by this compound:
Compound Name | Biological Activity | IC50 (µg/mL) | Notes |
---|---|---|---|
This compound | Antimicrobial | 15 (S. aureus) | Effective against Gram-positive bacteria |
5-(Carboxymethylene)-2,2-dimethyl-1,3-dioxane-4,6-dione | Anticancer | 25 (MCF-7) | Induces apoptosis |
Meldrum's Acid Derivatives | Antiviral | 12 (Influenza) | Broad-spectrum antiviral activity |
This table illustrates the diverse applications and efficacy of this compound compared to its analogs .
Properties
IUPAC Name |
5-(hydroxymethylidene)-2,2-dimethyl-1,3-dioxane-4,6-dione | |
---|---|---|
Source | PubChem | |
URL | https://pubchem.ncbi.nlm.nih.gov | |
Description | Data deposited in or computed by PubChem | |
InChI |
InChI=1S/C7H8O5/c1-7(2)11-5(9)4(3-8)6(10)12-7/h3,8H,1-2H3 | |
Source | PubChem | |
URL | https://pubchem.ncbi.nlm.nih.gov | |
Description | Data deposited in or computed by PubChem | |
InChI Key |
DELRMBDZSMPFPS-UHFFFAOYSA-N | |
Source | PubChem | |
URL | https://pubchem.ncbi.nlm.nih.gov | |
Description | Data deposited in or computed by PubChem | |
Canonical SMILES |
CC1(OC(=O)C(=CO)C(=O)O1)C | |
Source | PubChem | |
URL | https://pubchem.ncbi.nlm.nih.gov | |
Description | Data deposited in or computed by PubChem | |
Molecular Formula |
C7H8O5 | |
Source | PubChem | |
URL | https://pubchem.ncbi.nlm.nih.gov | |
Description | Data deposited in or computed by PubChem | |
DSSTOX Substance ID |
DTXSID00456523 | |
Record name | 5-(Hydroxymethylene)-2,2-dimethyl-1,3-dioxane-4,6-dione | |
Source | EPA DSSTox | |
URL | https://comptox.epa.gov/dashboard/DTXSID00456523 | |
Description | DSSTox provides a high quality public chemistry resource for supporting improved predictive toxicology. | |
Molecular Weight |
172.13 g/mol | |
Source | PubChem | |
URL | https://pubchem.ncbi.nlm.nih.gov | |
Description | Data deposited in or computed by PubChem | |
CAS No. |
15568-87-3 | |
Record name | 5-(Hydroxymethylene)-2,2-dimethyl-1,3-dioxane-4,6-dione | |
Source | EPA DSSTox | |
URL | https://comptox.epa.gov/dashboard/DTXSID00456523 | |
Description | DSSTox provides a high quality public chemistry resource for supporting improved predictive toxicology. | |
Synthesis routes and methods I
Procedure details
Synthesis routes and methods II
Procedure details
Retrosynthesis Analysis
AI-Powered Synthesis Planning: Our tool employs the Template_relevance Pistachio, Template_relevance Bkms_metabolic, Template_relevance Pistachio_ringbreaker, Template_relevance Reaxys, Template_relevance Reaxys_biocatalysis model, leveraging a vast database of chemical reactions to predict feasible synthetic routes.
One-Step Synthesis Focus: Specifically designed for one-step synthesis, it provides concise and direct routes for your target compounds, streamlining the synthesis process.
Accurate Predictions: Utilizing the extensive PISTACHIO, BKMS_METABOLIC, PISTACHIO_RINGBREAKER, REAXYS, REAXYS_BIOCATALYSIS database, our tool offers high-accuracy predictions, reflecting the latest in chemical research and data.
Strategy Settings
Precursor scoring | Relevance Heuristic |
---|---|
Min. plausibility | 0.01 |
Model | Template_relevance |
Template Set | Pistachio/Bkms_metabolic/Pistachio_ringbreaker/Reaxys/Reaxys_biocatalysis |
Top-N result to add to graph | 6 |
Feasible Synthetic Routes
Q1: The paper mentions condensation products of 1,2-diamines and hydroxymethylene malonic acid ester forming chelates with Cu(II) and Ni(II). Can you elaborate on how the introduction of coordinated ester groups, as opposed to acetyl or benzoyl oxygen, affects the properties of these metal complexes?
A1: The research demonstrates that replacing acetyl or benzoyl oxygen with coordinated ester groups in these chelates leads to a decrease in ligand field strength []. This observation suggests that the nature of the coordinating group significantly influences the electronic environment around the metal center. The weaker ligand field strength with coordinated ester groups could potentially impact the stability, reactivity, and magnetic properties of the resulting complexes. Further investigation into these specific effects would be needed to fully understand the implications of this structural change.
Disclaimer and Information on In-Vitro Research Products
Please be aware that all articles and product information presented on BenchChem are intended solely for informational purposes. The products available for purchase on BenchChem are specifically designed for in-vitro studies, which are conducted outside of living organisms. In-vitro studies, derived from the Latin term "in glass," involve experiments performed in controlled laboratory settings using cells or tissues. It is important to note that these products are not categorized as medicines or drugs, and they have not received approval from the FDA for the prevention, treatment, or cure of any medical condition, ailment, or disease. We must emphasize that any form of bodily introduction of these products into humans or animals is strictly prohibited by law. It is essential to adhere to these guidelines to ensure compliance with legal and ethical standards in research and experimentation.