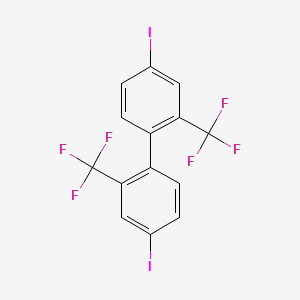
2,2'-Bis(trifluoromethyl)-4,4'-diiodobiphenyl
Overview
Description
2,2’-Bis(trifluoromethyl)-4,4’-diiodobiphenyl is an organic compound characterized by the presence of two trifluoromethyl groups and two iodine atoms attached to a biphenyl structure. This compound is notable for its unique chemical properties, which make it valuable in various scientific and industrial applications.
Preparation Methods
Synthetic Routes and Reaction Conditions: The synthesis of 2,2’-Bis(trifluoromethyl)-4,4’-diiodobiphenyl typically involves the following steps:
Starting Materials: The synthesis begins with the selection of appropriate starting materials, such as 2,2’-bis(trifluoromethyl)biphenyl.
Iodination Reaction: The introduction of iodine atoms is achieved through an iodination reaction. This can be done using iodine (I2) and an oxidizing agent like nitric acid (HNO3) or a halogen exchange reaction using iodine monochloride (ICl).
Reaction Conditions: The reaction is usually carried out under controlled temperature and pressure conditions to ensure the selective introduction of iodine atoms at the desired positions on the biphenyl ring.
Industrial Production Methods: In an industrial setting, the production of 2,2’-Bis(trifluoromethyl)-4,4’-diiodobiphenyl may involve large-scale iodination processes using continuous flow reactors. These reactors allow for precise control of reaction parameters, leading to higher yields and purity of the final product.
Chemical Reactions Analysis
Types of Reactions: 2,2’-Bis(trifluoromethyl)-4,4’-diiodobiphenyl can undergo various chemical reactions, including:
Substitution Reactions: The iodine atoms can be replaced by other functional groups through nucleophilic substitution reactions.
Oxidation and Reduction: The compound can participate in oxidation and reduction reactions, altering the oxidation state of the iodine atoms.
Coupling Reactions: The biphenyl structure allows for coupling reactions, forming larger, more complex molecules.
Common Reagents and Conditions:
Nucleophilic Substitution: Reagents such as sodium azide (NaN3) or potassium thiolate (KSR) can be used for substitution reactions.
Oxidation: Oxidizing agents like hydrogen peroxide (H2O2) or potassium permanganate (KMnO4) are commonly used.
Reduction: Reducing agents such as lithium aluminum hydride (LiAlH4) or sodium borohydride (NaBH4) can be employed.
Major Products: The major products formed from these reactions depend on the specific reagents and conditions used. For example, nucleophilic substitution with sodium azide can yield azido derivatives, while oxidation with hydrogen peroxide can produce iodinated biphenyl oxides.
Scientific Research Applications
2,2’-Bis(trifluoromethyl)-4,4’-diiodobiphenyl has a wide range of applications in scientific research, including:
Chemistry: It is used as a building block for the synthesis of more complex organic molecules and polymers.
Biology: The compound can be used in the development of bioactive molecules and as a probe in biochemical assays.
Industry: The compound is used in the production of specialty chemicals and materials with unique properties, such as high thermal stability and resistance to chemical degradation.
Mechanism of Action
The mechanism of action of 2,2’-Bis(trifluoromethyl)-4,4’-diiodobiphenyl involves its interaction with specific molecular targets and pathways. The trifluoromethyl groups enhance the compound’s lipophilicity, allowing it to easily penetrate cell membranes. The iodine atoms can participate in halogen bonding, influencing the compound’s binding affinity to target proteins and enzymes. These interactions can modulate various biochemical pathways, leading to the compound’s observed effects.
Comparison with Similar Compounds
2,2’-Bis(trifluoromethyl)-4,4’-diaminobiphenyl: This compound has amino groups instead of iodine atoms, leading to different reactivity and applications.
2,2’-Bis(trifluoromethyl)-4,4’-dinitrobiphenyl: The presence of nitro groups imparts distinct electronic properties and reactivity.
2,2’-Bis(trifluoromethyl)-4,4’-dibromobiphenyl: Bromine atoms replace iodine, resulting in variations in chemical behavior and applications.
Uniqueness: 2,2’-Bis(trifluoromethyl)-4,4’-diiodobiphenyl is unique due to the combination of trifluoromethyl and iodine substituents. This combination imparts specific electronic and steric effects, making the compound valuable for specialized applications in organic synthesis, materials science, and medicinal chemistry.
Properties
IUPAC Name |
4-iodo-1-[4-iodo-2-(trifluoromethyl)phenyl]-2-(trifluoromethyl)benzene | |
---|---|---|
Source | PubChem | |
URL | https://pubchem.ncbi.nlm.nih.gov | |
Description | Data deposited in or computed by PubChem | |
InChI |
InChI=1S/C14H6F6I2/c15-13(16,17)11-5-7(21)1-3-9(11)10-4-2-8(22)6-12(10)14(18,19)20/h1-6H | |
Source | PubChem | |
URL | https://pubchem.ncbi.nlm.nih.gov | |
Description | Data deposited in or computed by PubChem | |
InChI Key |
OQUYDTUCILYGGP-UHFFFAOYSA-N | |
Source | PubChem | |
URL | https://pubchem.ncbi.nlm.nih.gov | |
Description | Data deposited in or computed by PubChem | |
Canonical SMILES |
C1=CC(=C(C=C1I)C(F)(F)F)C2=C(C=C(C=C2)I)C(F)(F)F | |
Source | PubChem | |
URL | https://pubchem.ncbi.nlm.nih.gov | |
Description | Data deposited in or computed by PubChem | |
Molecular Formula |
C14H6F6I2 | |
Source | PubChem | |
URL | https://pubchem.ncbi.nlm.nih.gov | |
Description | Data deposited in or computed by PubChem | |
DSSTOX Substance ID |
DTXSID20371163 | |
Record name | 4,4'-Diiodo-2,2'-bis(trifluoromethyl)-1,1'-biphenyl | |
Source | EPA DSSTox | |
URL | https://comptox.epa.gov/dashboard/DTXSID20371163 | |
Description | DSSTox provides a high quality public chemistry resource for supporting improved predictive toxicology. | |
Molecular Weight |
542.00 g/mol | |
Source | PubChem | |
URL | https://pubchem.ncbi.nlm.nih.gov | |
Description | Data deposited in or computed by PubChem | |
CAS No. |
89803-70-3 | |
Record name | 4,4'-Diiodo-2,2'-bis(trifluoromethyl)-1,1'-biphenyl | |
Source | EPA DSSTox | |
URL | https://comptox.epa.gov/dashboard/DTXSID20371163 | |
Description | DSSTox provides a high quality public chemistry resource for supporting improved predictive toxicology. | |
Synthesis routes and methods
Procedure details
Retrosynthesis Analysis
AI-Powered Synthesis Planning: Our tool employs the Template_relevance Pistachio, Template_relevance Bkms_metabolic, Template_relevance Pistachio_ringbreaker, Template_relevance Reaxys, Template_relevance Reaxys_biocatalysis model, leveraging a vast database of chemical reactions to predict feasible synthetic routes.
One-Step Synthesis Focus: Specifically designed for one-step synthesis, it provides concise and direct routes for your target compounds, streamlining the synthesis process.
Accurate Predictions: Utilizing the extensive PISTACHIO, BKMS_METABOLIC, PISTACHIO_RINGBREAKER, REAXYS, REAXYS_BIOCATALYSIS database, our tool offers high-accuracy predictions, reflecting the latest in chemical research and data.
Strategy Settings
Precursor scoring | Relevance Heuristic |
---|---|
Min. plausibility | 0.01 |
Model | Template_relevance |
Template Set | Pistachio/Bkms_metabolic/Pistachio_ringbreaker/Reaxys/Reaxys_biocatalysis |
Top-N result to add to graph | 6 |
Feasible Synthetic Routes
Disclaimer and Information on In-Vitro Research Products
Please be aware that all articles and product information presented on BenchChem are intended solely for informational purposes. The products available for purchase on BenchChem are specifically designed for in-vitro studies, which are conducted outside of living organisms. In-vitro studies, derived from the Latin term "in glass," involve experiments performed in controlled laboratory settings using cells or tissues. It is important to note that these products are not categorized as medicines or drugs, and they have not received approval from the FDA for the prevention, treatment, or cure of any medical condition, ailment, or disease. We must emphasize that any form of bodily introduction of these products into humans or animals is strictly prohibited by law. It is essential to adhere to these guidelines to ensure compliance with legal and ethical standards in research and experimentation.