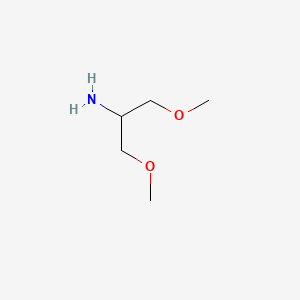
1,3-Dimethoxypropan-2-amine
Overview
Description
1,3-Dimethoxypropan-2-amine (CAS 78531-29-0) is a secondary amine with the molecular formula C₅H₁₃NO₂ and a molecular weight of 119.16 g/mol. Key properties include:
Preparation Methods
Detailed Synthetic Routes and Reaction Conditions
Amination of Methoxy-Substituted Precursors
One common approach is the amination of 1,3-dimethoxypropan derivatives, such as 1,3-dichloropropan-2-ol or 1,3-dimethoxypropane oxide, using ammonia or ammonium salts. This method typically proceeds under controlled temperature and solvent conditions to minimize side reactions.
- Reaction conditions: Temperature range 60–80°C; solvents such as methanol or tetrahydrofuran (THF)
- Catalysts: Often none required; sometimes basic conditions (e.g., potassium carbonate) to facilitate substitution
- Outcome: High selectivity for the amine product with minimal over-alkylation
Alkylation of Glycerol Derivatives
This method involves the alkylation of glycerol or its derivatives with methoxide ions to introduce methoxy groups, followed by amination.
- Key reagents: 1,3-dichloropropan-2-ol, potassium methoxide
- Conditions: Basic medium, moderate temperatures (~65°C)
- Advantages: Straightforward, uses readily available starting materials
Catalytic Hydrogenation of Nitriles
Hydrogenation of 1,3-dimethoxypropanenitrile under catalytic conditions yields the target amine.
- Catalysts: Raney nickel, palladium on carbon
- Conditions: Temperature 50–100°C, hydrogen pressure 5–10 bar
- Benefits: High purity product, scalable for industrial production
Ring-Opening Amination of Epoxides
The ring-opening of 1,3-dimethoxypropane oxide with ammonia provides a direct route to the amine.
- Conditions: Aqueous or solvent-free, mild temperatures
- Selectivity: High, with minimal side products
Industrial Scale Synthesis and Process Optimization
Industrial production emphasizes large-scale reactors, inert atmospheres (nitrogen or argon), and precise temperature control (2–8°C) to prevent side reactions and ensure product purity. Inline monitoring techniques such as FTIR spectroscopy are employed to track reaction progress and adjust reagent stoichiometry in real time.
Table 1: Representative Industrial Synthesis Parameters
Parameter | Typical Range/Value | Notes |
---|---|---|
Reactor volume | 10 L to 3000 L | Scalable from pilot to production scale |
Temperature | 2–8°C (reaction), 60–80°C (amination) | Low temp to avoid side reactions |
Solvent | Methanol, THF | Methanol enhances solubility |
Catalyst | None or Raney Ni/Pd (hydrogenation) | Depends on route |
Reaction time | 8–40 hours | Longer times for complete conversion |
Purification | Vacuum drying, recrystallization | Ensures high purity (>97%) |
Purification and Characterization
Post-synthesis purification typically involves vacuum drying and recrystallization from solvents such as ethyl acetate or hexane to achieve purity levels above 97%. Analytical techniques used to confirm structural integrity include:
Nuclear Magnetic Resonance (NMR):
- ^1H NMR: Methoxy protons at δ 3.3–3.5 ppm, amine-bearing methylene at δ 2.6–2.8 ppm
- ^13C NMR: Methoxy carbons at 50–55 ppm, amine carbon at 35–40 ppm
-
- N-H stretch at 3300–3500 cm⁻¹
- C-O-C stretch at 1100–1250 cm⁻¹
-
- Molecular ion peak at m/z 119.16 consistent with C5H13NO2
Research Findings on Reaction Parameters
Effect of Solvent Polarity and pH
- Polar aprotic solvents (e.g., DMF) increase nucleophilicity of the amine, enhancing substitution reaction rates.
- Protic solvents (e.g., ethanol) may reduce reactivity due to hydrogen bonding.
- Alkaline pH (>10) favors deprotonated amine forms, accelerating nucleophilic substitution.
- Acidic pH (<8) leads to protonation, reducing nucleophilicity and increasing side reactions.
Computational and Experimental Insights on Stability
- Density Functional Theory (DFT) calculations indicate N-oxide formation as a low-energy degradation pathway under oxidative conditions.
- UV irradiation can cause demethylation, producing formaldehyde and 3-aminopropane-1,2-diol.
- Experimental validation via HPLC-MS confirms intermediates consistent with these pathways.
Summary Table of Preparation Methods
Method | Key Reagents/Conditions | Advantages | Limitations | Industrial Use |
---|---|---|---|---|
Amination of methoxy precursors | Ammonia, methanol/THF, 60–80°C | High selectivity, mild conditions | Requires pure precursors | Widely used |
Alkylation of glycerol derivatives | 1,3-dichloropropan-2-ol, K methoxide, base | Readily available materials | Side reactions possible | Common in lab scale |
Catalytic hydrogenation of nitriles | Raney Ni/Pd, H2, 50–100°C, 5–10 bar | High purity, scalable | Requires high-pressure equipment | Preferred for large scale |
Ring-opening amination of epoxides | 1,3-dimethoxypropane oxide, NH3, mild temp | Direct, efficient | Epoxide availability | Used in specialty synthesis |
Chemical Reactions Analysis
Types of Reactions
1,3-Dimethoxypropan-2-amine undergoes various chemical reactions, including:
Oxidation: It can be oxidized to form corresponding oxides.
Reduction: It can be reduced to form amines.
Substitution: It can undergo nucleophilic substitution reactions.
Common Reagents and Conditions
Oxidation: Common oxidizing agents include potassium permanganate and hydrogen peroxide.
Reduction: Reducing agents such as lithium aluminum hydride and sodium borohydride are used.
Substitution: Nucleophiles such as halides and alkoxides are commonly used in substitution reactions.
Major Products
Oxidation: Produces corresponding oxides.
Reduction: Produces primary amines.
Substitution: Produces substituted amines and ethers.
Scientific Research Applications
Organic Synthesis
1,3-Dimethoxypropan-2-amine serves as a valuable reagent in organic synthesis. Its reactive functional groups allow it to participate in various chemical reactions, including:
- Nucleophilic Substitution : Acts as a nucleophile to form new chemical bonds.
- Oxidation and Reduction : Can be oxidized to corresponding oxides or reduced to primary amines, facilitating the synthesis of complex organic molecules.
Medicinal Chemistry
Research has indicated potential therapeutic applications of this compound:
- Enzyme Inhibition : It has been shown to inhibit dihydroorotate dehydrogenase (DHODH), an enzyme involved in pyrimidine synthesis. This inhibition can reduce nucleotide synthesis crucial for cell proliferation, making it a candidate for treating autoimmune diseases and certain cancers.
- Anticancer Activity : In vitro studies have demonstrated that this compound exhibits cytotoxic effects against various cancer cell lines. For instance, it has been observed to decrease the viability of leukemia cells by promoting differentiation markers indicative of reduced malignancy.
Biological Studies
The compound's structure suggests potential interactions with biological systems:
- Interaction with Enzymes and Receptors : The presence of methoxy groups may enhance solubility and reactivity, allowing it to function as a ligand that modulates biological pathways by binding to specific sites.
Case Studies
The following table summarizes significant findings from relevant studies on this compound:
Study | Findings | Implications |
---|---|---|
Sykes et al. (2017) | Demonstrated DHODH inhibition leads to reduced leukemic stem cells in vitro. | Suggests potential for this compound in leukemia treatment. |
Reis et al. (2017) | Investigated DHODH inhibitors' role in autoimmune diseases. | Supports further exploration of this compound for therapeutic applications in autoimmune conditions. |
Mechanism of Action
The mechanism of action of 1,3-dimethoxypropan-2-amine involves its interaction with molecular targets such as enzymes and receptors. It acts as a nucleophile in substitution reactions, where it donates an electron pair to form new chemical bonds. The pathways involved include nucleophilic substitution and amination reactions .
Comparison with Similar Compounds
Comparison with Structurally Similar Compounds
Key Compounds for Comparison
The following compounds were selected for comparison based on structural and functional similarities:
Compound Name | CAS Number | Molecular Formula | Molecular Weight (g/mol) | Boiling Point (°C) | Density (g/cm³) | pKa | Hazard Class | UN ID |
---|---|---|---|---|---|---|---|---|
1,3-Dimethoxypropan-2-amine | 78531-29-0 | C₅H₁₃NO₂ | 119.16 | 160.8 ± 25.0 | 0.932 ± 0.06 | 7.00 | 8 | 2735 |
Dimethylaminopropylamine | 109-55-7 | C₅H₁₄N₂ | 114.19 | Not reported | Not reported | Not reported | 8 | 2734 |
3-(2'-Methoxyethoxy)propylamine | 54303-31-0 | C₆H₁₅NO₂ | 133.19 | Not reported | Not reported | Not reported | Not reported | Not reported |
3,3-Dimethoxypropan-1-amine | 60185-84-4 | C₅H₁₃NO₂ | 119.16 | Not reported | Not reported | Not reported | Not reported | Not reported |
N,N-Dibenzyl-1,3-dimethoxypropan-2-amine | 1156542-80-1 | C₁₉H₂₅NO₂ | 299.41 | Not reported | Not reported | Not reported | Not reported | Not reported |
Structural and Functional Analysis
This compound vs. Dimethylaminopropylamine (CAS 109-55-7)
- Structural Differences: Dimethylaminopropylamine has a linear propane backbone with two methyl groups on the central nitrogen atom (N,N-dimethyl-1,3-propanediamine), whereas this compound features methoxy groups on carbons 1 and 3 and an amine on carbon 2.
- Hazard Profile : Both compounds are classified under hazard class 8 (corrosive), but their UN IDs differ (2735 vs. 2734), indicating distinct regulatory handling requirements .
This compound vs. 3,3-Dimethoxypropan-1-amine (CAS 60185-84-4)
- Isomerism: These compounds are structural isomers.
- Applications : While this compound is used in specialty chemical synthesis (e.g., as a chiral auxiliary), 3,3-dimethoxypropan-1-amine’s applications are less documented .
This compound vs. N,N-Dibenzyl-1,3-dimethoxypropan-2-amine (CAS 1156542-80-1)
- Derivative Comparison : The dibenzyl derivative has a significantly higher molecular weight (299.41 g/mol) due to benzyl substituents. This modification enhances steric bulk, making it more suitable for pharmaceutical intermediates but less volatile .
Physicochemical Property Trends
- Boiling Points : The target compound’s boiling point (~160°C) is moderately high compared to aliphatic amines, likely due to hydrogen bonding from the methoxy groups. Derivatives with larger substituents (e.g., benzyl groups) exhibit higher boiling points but lack reported data .
- pKa Values : The pKa of 7.00 for this compound suggests weak basicity, comparable to other secondary amines. Methoxy groups may slightly decrease basicity by electron-withdrawing effects .
Hazard and Handling Considerations
- Corrosivity: Both this compound and dimethylaminopropylamine are corrosive (hazard class 8), requiring inert storage conditions .
- Volatility : The target compound’s vapor pressure (2.35 mmHg at 25°C) indicates moderate volatility, necessitating ventilation during handling .
Biological Activity
1,3-Dimethoxypropan-2-amine (1,3-DMPA) is a chemical compound characterized by a central amine group flanked by two methoxy functional groups. Its unique structure suggests potential biological activities that merit investigation. This article aims to provide a comprehensive overview of the biological activity of 1,3-DMPA, including its mechanisms of action, potential therapeutic applications, and relevant case studies.
1,3-DMPA has the molecular formula and can be represented structurally as follows:
The presence of two methoxy groups may influence its interaction with biological systems, potentially enhancing its solubility and reactivity.
The biological activity of 1,3-DMPA is hypothesized to involve interactions with various molecular targets such as enzymes and receptors. It may function as a ligand that modulates biological pathways by binding to specific sites. Research indicates that compounds with similar structures often affect neurotransmitter systems, suggesting that 1,3-DMPA could have implications in neuropharmacology .
Inhibition of Enzymatic Activity
Research has identified that 1,3-DMPA may inhibit dihydroorotate dehydrogenase (DHODH), an enzyme critical in pyrimidine synthesis. This inhibition can lead to decreased nucleotide synthesis, which is essential for cell proliferation. DHODH inhibitors have been explored for their potential in treating autoimmune diseases and certain cancers .
Anticancer Activity
In vitro studies have shown that 1,3-DMPA exhibits cytotoxic effects against various cancer cell lines. For example, it has been documented to reduce the viability of leukemia cells by promoting differentiation markers indicative of reduced malignancy .
Case Studies
Study | Findings | Implications |
---|---|---|
Sykes et al. (2017) | Demonstrated that DHODH inhibition leads to reduced leukemic stem cells in vitro. | Suggests potential for 1,3-DMPA in leukemia treatment. |
Reis et al. (2017) | Investigated the role of DHODH inhibitors in autoimmune diseases. | Supports further exploration of 1,3-DMPA for therapeutic applications in autoimmune conditions. |
Research Applications
1,3-DMPA is being studied across multiple domains:
- Medicinal Chemistry : As a potential therapeutic agent targeting specific pathways involved in cancer and autoimmune diseases.
- Organic Synthesis : Utilized as an intermediate in the synthesis of various organic compounds due to its reactive functional groups.
Q & A
Basic Research Questions
Q. What are the optimal synthetic routes for 1,3-Dimethoxypropan-2-amine, and how do reaction conditions influence yield and purity?
Answer: this compound (CAS 78531-29-0, C₅H₁₃NO₂) can be synthesized via alkylation or ammoniation. Key methods include:
- Alkylation of glycerol derivatives : Reacting 1,3-dichloropropan-2-ol with methoxide ions under controlled basic conditions (e.g., K₂CO₃) to introduce methoxy groups, followed by amination .
- Hydrogenation of nitriles : Catalytic hydrogenation of 1,3-dimethoxypropanenitrile using Raney nickel or palladium catalysts at 50–100°C and 5–10 bar H₂ pressure .
- Amination of epoxides : Ring-opening of 1,3-dimethoxypropane oxide with ammonia under aqueous or solvent-free conditions .
Critical parameters :
- Temperature control (60–80°C) minimizes side reactions like over-alkylation.
- Solvent choice (e.g., methanol vs. THF) affects reaction kinetics and purity. Methanol enhances solubility of intermediates but may require longer reaction times .
Q. How can researchers confirm the structural integrity of this compound using spectroscopic methods?
Answer: Key analytical techniques :
- ¹H/¹³C NMR :
- ¹H NMR : Peaks at δ 3.3–3.5 ppm (methoxy groups), δ 2.6–2.8 ppm (CH₂NH₂), and δ 1.8–2.0 ppm (central CH group) .
- ¹³C NMR : Signals at 50–55 ppm (methoxy carbons) and 35–40 ppm (amine-bearing carbon) .
- IR spectroscopy : Strong absorption at 3300–3500 cm⁻¹ (N-H stretch) and 1100–1250 cm⁻¹ (C-O-C stretch) .
- Mass spectrometry : Molecular ion peak at m/z 119.16 (M⁺) with fragmentation patterns consistent with methoxy and amine groups .
Validation : Compare spectral data with PubChem records (InChI Key: DPLCQNGJZJXXPM-UHFFFAOYSA-N) to resolve ambiguities .
Advanced Research Questions
Q. How do solvent polarity and pH influence the reactivity of this compound in nucleophilic substitution reactions?
Answer: The compound’s reactivity is modulated by:
- Solvent polarity : Polar aprotic solvents (e.g., DMF) enhance nucleophilicity of the amine by stabilizing transition states. In contrast, protic solvents (e.g., ethanol) reduce reactivity via hydrogen bonding .
- pH : At pH > 10, the amine exists predominantly in its deprotonated form (NH⁻), accelerating nucleophilic attacks. Below pH 8, protonation reduces reactivity, favoring side reactions like hydrolysis .
Case study : In Suzuki-Miyaura coupling, optimized yields (>85%) were achieved using DMF (ε = 37) and pH 11–12 .
Q. How can computational modeling resolve contradictions in reported degradation pathways of this compound under oxidative conditions?
Answer: Conflicting studies suggest two degradation pathways:
N-Oxide formation : Predicted via DFT calculations to have a lower activation energy (ΔG‡ = 45 kJ/mol) in the presence of O₂ .
Demethylation : Favored under UV irradiation, producing formaldehyde and 3-aminopropane-1,2-diol .
Methodology :
- Perform ab initio molecular dynamics (AIMD) to simulate reaction trajectories under varying O₂ concentrations.
- Validate with HPLC-MS to detect intermediates like N-oxide (observed m/z 135.1) .
Q. What experimental strategies mitigate batch-to-batch variability in this compound synthesis for reproducible pharmacological studies?
Answer: Critical factors :
- Raw material purity : Use HPLC-grade 1,3-dichloropropan-2-ol (≥99%) to minimize halogenated byproducts .
- Process control : Implement inline FTIR monitoring to track methoxy group incorporation and adjust reagent stoichiometry in real time .
- Post-synthesis purification : Employ fractional distillation (BP 98–100°C) or recrystallization from ethyl acetate/hexane mixtures .
Case study : A GMP-compliant facility reduced variability (<2% RSD) by standardizing reaction conditions (T = 65°C, 8 hr) and using automated pH control .
Q. How does the stereoelectronic profile of this compound influence its coordination chemistry with transition metals?
Answer: The compound’s methoxy groups act as weak σ-donors, while the amine serves as a stronger Lewis base. Key interactions:
- Cu(II) complexes : Square-planar geometry with two amine ligands and two methoxy oxygens (λmax = 600–650 nm) .
- Fe(III) complexes : Octahedral coordination, but methoxy groups reduce stability due to steric hindrance (log β = 4.2 vs. 5.8 for unsubstituted analogs) .
Methodology :
- X-ray crystallography : Resolve bonding modes (e.g., monodentate vs. bidentate).
- Cyclic voltammetry : Compare redox potentials to assess electronic effects .
Properties
IUPAC Name |
1,3-dimethoxypropan-2-amine | |
---|---|---|
Source | PubChem | |
URL | https://pubchem.ncbi.nlm.nih.gov | |
Description | Data deposited in or computed by PubChem | |
InChI |
InChI=1S/C5H13NO2/c1-7-3-5(6)4-8-2/h5H,3-4,6H2,1-2H3 | |
Source | PubChem | |
URL | https://pubchem.ncbi.nlm.nih.gov | |
Description | Data deposited in or computed by PubChem | |
InChI Key |
DSZCUJHVOLGWBO-UHFFFAOYSA-N | |
Source | PubChem | |
URL | https://pubchem.ncbi.nlm.nih.gov | |
Description | Data deposited in or computed by PubChem | |
Canonical SMILES |
COCC(COC)N | |
Source | PubChem | |
URL | https://pubchem.ncbi.nlm.nih.gov | |
Description | Data deposited in or computed by PubChem | |
Molecular Formula |
C5H13NO2 | |
Source | PubChem | |
URL | https://pubchem.ncbi.nlm.nih.gov | |
Description | Data deposited in or computed by PubChem | |
DSSTOX Substance ID |
DTXSID70363790 | |
Record name | 1,3-dimethoxypropan-2-amine | |
Source | EPA DSSTox | |
URL | https://comptox.epa.gov/dashboard/DTXSID70363790 | |
Description | DSSTox provides a high quality public chemistry resource for supporting improved predictive toxicology. | |
Molecular Weight |
119.16 g/mol | |
Source | PubChem | |
URL | https://pubchem.ncbi.nlm.nih.gov | |
Description | Data deposited in or computed by PubChem | |
CAS No. |
78531-29-0 | |
Record name | 1,3-dimethoxypropan-2-amine | |
Source | EPA DSSTox | |
URL | https://comptox.epa.gov/dashboard/DTXSID70363790 | |
Description | DSSTox provides a high quality public chemistry resource for supporting improved predictive toxicology. | |
Record name | 1,3-dimethoxypropan-2-amine | |
Source | European Chemicals Agency (ECHA) | |
URL | https://echa.europa.eu/information-on-chemicals | |
Description | The European Chemicals Agency (ECHA) is an agency of the European Union which is the driving force among regulatory authorities in implementing the EU's groundbreaking chemicals legislation for the benefit of human health and the environment as well as for innovation and competitiveness. | |
Explanation | Use of the information, documents and data from the ECHA website is subject to the terms and conditions of this Legal Notice, and subject to other binding limitations provided for under applicable law, the information, documents and data made available on the ECHA website may be reproduced, distributed and/or used, totally or in part, for non-commercial purposes provided that ECHA is acknowledged as the source: "Source: European Chemicals Agency, http://echa.europa.eu/". Such acknowledgement must be included in each copy of the material. ECHA permits and encourages organisations and individuals to create links to the ECHA website under the following cumulative conditions: Links can only be made to webpages that provide a link to the Legal Notice page. | |
Retrosynthesis Analysis
AI-Powered Synthesis Planning: Our tool employs the Template_relevance Pistachio, Template_relevance Bkms_metabolic, Template_relevance Pistachio_ringbreaker, Template_relevance Reaxys, Template_relevance Reaxys_biocatalysis model, leveraging a vast database of chemical reactions to predict feasible synthetic routes.
One-Step Synthesis Focus: Specifically designed for one-step synthesis, it provides concise and direct routes for your target compounds, streamlining the synthesis process.
Accurate Predictions: Utilizing the extensive PISTACHIO, BKMS_METABOLIC, PISTACHIO_RINGBREAKER, REAXYS, REAXYS_BIOCATALYSIS database, our tool offers high-accuracy predictions, reflecting the latest in chemical research and data.
Strategy Settings
Precursor scoring | Relevance Heuristic |
---|---|
Min. plausibility | 0.01 |
Model | Template_relevance |
Template Set | Pistachio/Bkms_metabolic/Pistachio_ringbreaker/Reaxys/Reaxys_biocatalysis |
Top-N result to add to graph | 6 |
Feasible Synthetic Routes
Disclaimer and Information on In-Vitro Research Products
Please be aware that all articles and product information presented on BenchChem are intended solely for informational purposes. The products available for purchase on BenchChem are specifically designed for in-vitro studies, which are conducted outside of living organisms. In-vitro studies, derived from the Latin term "in glass," involve experiments performed in controlled laboratory settings using cells or tissues. It is important to note that these products are not categorized as medicines or drugs, and they have not received approval from the FDA for the prevention, treatment, or cure of any medical condition, ailment, or disease. We must emphasize that any form of bodily introduction of these products into humans or animals is strictly prohibited by law. It is essential to adhere to these guidelines to ensure compliance with legal and ethical standards in research and experimentation.