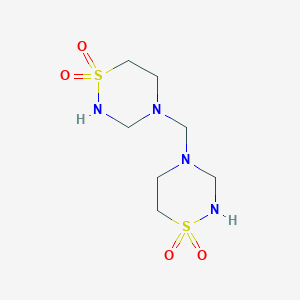
Taurolidine
Overview
Description
Taurolidine is an antimicrobial compound derived from the amino acid taurine. It was first synthesized in the 1970s and has been primarily used for the prevention of catheter-related infections. This compound exhibits broad-spectrum antimicrobial activity against both Gram-positive and Gram-negative bacteria, as well as certain fungi and mycobacteria .
Mechanism of Action
Target of Action
Taurolidine is a broad-spectrum antimicrobial agent that primarily targets lipopolysaccharides (LPS) . It has activity against both Gram-positive and Gram-negative bacteria, in addition to mycobacteria and certain strains of fungi . It also targets circulating tumor cells, which correlate negatively with disease-free survival and overall survival .
Mode of Action
The mechanism of action of this compound and its active metabolites is non-specific and involves the irreversible binding of its methylol groups to microbial cell walls . This results in a subsequent loss of cell wall integrity and eventual cell death . In addition, it appears to reduce bacterial adhesion to mammalian cells and neutralize bacterial endo- and exotoxins .
Biochemical Pathways
This compound’s action involves the metabolism of this compound to taurinamide and ultimately taurine and water, liberating methylol groups that chemically react with the mureins in the bacterial cell wall and with the amino and hydroxyl groups of endotoxins and exotoxins . This reaction denatures the endotoxins and complex polysaccharide and lipopolysaccharide components of the bacterial cell wall and inactivates susceptible exotoxins . This compound also induces autophagy, which augments both host resistance and disease tolerance to bacterial infection .
Pharmacokinetics
This compound is metabolized rapidly via the metabolites taurultam and methylol taurinamide, which also have a bactericidal action, to taurine, an endogenous aminosulphonic acid, carbon dioxide, and water . The this compound metabolite taurultam demonstrated a shorter half-life and lower systemic exposure than taurinamide .
Result of Action
The result of this compound’s action is the prevention of infections in catheters . It decreases the adherence of bacteria and fungi to host cells by destructing the fimbriae and flagella, thus preventing biofilm formation . It also enhances apoptosis, inhibits angiogenesis, reduces tumor adherence, downregulates pro-inflammatory cytokine release, and stimulates anticancer immune regulation following surgical trauma .
Action Environment
In a biological environment, this compound exists in equilibrium with taurultam derivatives . This equilibrium is described theoretically as a 2-step process without an energy barrier: formation of cationic this compound followed by a nucleophilic attack of O(hydroxyl) on the exocyclic C(methylene) . This compound’s effectiveness against bacterial infections at laparoscopic lesions may be due to its ready availability, as it is administered in high doses after peritonitis surgery .
Biochemical Analysis
Biochemical Properties
Taurolidine interacts with various biomolecules in its role as an antimicrobial agent. Its putative method of action involves the metabolism of this compound to taurinamide and ultimately taurine and water, liberating formaldehyde that chemically reacts with the mureins in the bacterial cell wall and with the amino and hydroxyl groups of endotoxins and exotoxins . This reaction denatures the endotoxins and complex polysaccharide and lipopolysaccharide components of the bacterial cell wall and inactivates susceptible exotoxins .
Cellular Effects
This compound has been shown to have various effects on cells. It decreases the adherence of bacteria and fungi to host cells by destructing the fimbriae and flagella, thus preventing biofilm formation . It also induces cancer cell death through a variety of mechanisms, including enhancement of apoptosis, inhibition of angiogenesis, reduction of tumor adherence, downregulation of pro-inflammatory cytokine release, and stimulation of anticancer immune regulation .
Molecular Mechanism
The molecular mechanism of this compound involves the irreversible binding of its methylol groups to microbial cell walls, resulting in a subsequent loss of cell wall integrity and eventual cell death . In addition, it appears to reduce bacterial adhesion to mammalian cells and neutralize bacterial endo- and exotoxins .
Temporal Effects in Laboratory Settings
In laboratory settings, this compound has been observed to cause liver injury after short-term use in in vitro and in vivo models, probably due to direct toxic effects on hepatocytes . This suggests that the effects of this compound can change over time and that its stability, degradation, and long-term effects on cellular function need to be carefully monitored in laboratory studies.
Dosage Effects in Animal Models
In animal models of osteosarcoma, this compound was administered at different concentrations. Body weight was significantly lower in the group treated with a higher concentration of this compound, consistent with elevated mortality in this group . This suggests that the effects of this compound can vary with different dosages and that high doses may have toxic or adverse effects.
Metabolic Pathways
This compound is metabolized rapidly in the body via the metabolites taurultam and methylol taurinamide, which also have a bactericidal action, to taurine, an endogenous aminosulphonic acid, carbon dioxide, and water . This suggests that this compound is involved in metabolic pathways that interact with enzymes or cofactors and can affect metabolic flux or metabolite levels.
Preparation Methods
Synthetic Routes and Reaction Conditions: The synthesis of taurolidine involves the reaction of taurine with formaldehyde. One of the early methods described involves reacting taurine with formaldehyde in the presence of hydrochloric acid to form taurinamide, which is then further reacted with formaldehyde to produce this compound .
Industrial Production Methods: Industrial production of this compound often involves the use of phthalic anhydride as a protecting group for the amine functionality of taurine. This intermediate is then reacted with formaldehyde to produce this compound. The process requires careful control of reaction conditions to ensure high purity and yield .
Chemical Reactions Analysis
Types of Reactions: Taurolidine undergoes several types of chemical reactions, including hydrolysis and decomposition. In aqueous solutions, this compound rapidly hydrolyzes to form taurultam and methylol-taurultam, which further decompose to taurinamide and methylol-taurinamide .
Common Reagents and Conditions:
Hydrolysis: Water is the primary reagent, and the reaction occurs readily at physiological pH.
Decomposition: The decomposition products are formed under mild conditions, typically in aqueous environments.
Major Products: The major products of this compound hydrolysis and decomposition are taurultam, methylol-taurultam, taurinamide, and methylol-taurinamide .
Scientific Research Applications
Taurolidine has been extensively studied for its antimicrobial properties and its potential use in various medical applications:
Infection Prevention: this compound is used as a catheter lock solution to prevent catheter-related bloodstream infections
Cancer Therapy: Research has shown that this compound exhibits antineoplastic activity, making it a potential candidate for cancer treatment
Surgical Applications: this compound irrigation has been used to reduce surgical site infections in orthopedic surgeries.
Antiadhesive Properties: this compound inhibits the adhesion of bacteria to epithelial and fibroblast cells, reducing the risk of biofilm formation.
Comparison with Similar Compounds
Taurultam: A hydrolysis product of taurolidine with similar antimicrobial properties.
Methylol-taurultam: Another decomposition product with antimicrobial activity.
1,1-Dioxothiane: A structurally related compound with similar bond lengths and antimicrobial properties.
Uniqueness: this compound’s unique mechanism of action, involving the release of methylol groups, sets it apart from other antimicrobial agents. Its ability to prevent bacterial adhesion and neutralize toxins makes it particularly effective in preventing infections in medical settings .
Properties
IUPAC Name |
4-[(1,1-dioxo-1,2,4-thiadiazinan-4-yl)methyl]-1,2,4-thiadiazinane 1,1-dioxide | |
---|---|---|
Source | PubChem | |
URL | https://pubchem.ncbi.nlm.nih.gov | |
Description | Data deposited in or computed by PubChem | |
InChI |
InChI=1S/C7H16N4O4S2/c12-16(13)3-1-10(5-8-16)7-11-2-4-17(14,15)9-6-11/h8-9H,1-7H2 | |
Source | PubChem | |
URL | https://pubchem.ncbi.nlm.nih.gov | |
Description | Data deposited in or computed by PubChem | |
InChI Key |
AJKIRUJIDFJUKJ-UHFFFAOYSA-N | |
Source | PubChem | |
URL | https://pubchem.ncbi.nlm.nih.gov | |
Description | Data deposited in or computed by PubChem | |
Canonical SMILES |
C1CS(=O)(=O)NCN1CN2CCS(=O)(=O)NC2 | |
Source | PubChem | |
URL | https://pubchem.ncbi.nlm.nih.gov | |
Description | Data deposited in or computed by PubChem | |
Molecular Formula |
C7H16N4O4S2 | |
Source | PubChem | |
URL | https://pubchem.ncbi.nlm.nih.gov | |
Description | Data deposited in or computed by PubChem | |
DSSTOX Substance ID |
DTXSID00173001 | |
Record name | Taurolidine | |
Source | EPA DSSTox | |
URL | https://comptox.epa.gov/dashboard/DTXSID00173001 | |
Description | DSSTox provides a high quality public chemistry resource for supporting improved predictive toxicology. | |
Molecular Weight |
284.4 g/mol | |
Source | PubChem | |
URL | https://pubchem.ncbi.nlm.nih.gov | |
Description | Data deposited in or computed by PubChem | |
CAS No. |
19388-87-5 | |
Record name | Taurolidine | |
Source | CAS Common Chemistry | |
URL | https://commonchemistry.cas.org/detail?cas_rn=19388-87-5 | |
Description | CAS Common Chemistry is an open community resource for accessing chemical information. Nearly 500,000 chemical substances from CAS REGISTRY cover areas of community interest, including common and frequently regulated chemicals, and those relevant to high school and undergraduate chemistry classes. This chemical information, curated by our expert scientists, is provided in alignment with our mission as a division of the American Chemical Society. | |
Explanation | The data from CAS Common Chemistry is provided under a CC-BY-NC 4.0 license, unless otherwise stated. | |
Record name | Taurolidine [INN:BAN] | |
Source | ChemIDplus | |
URL | https://pubchem.ncbi.nlm.nih.gov/substance/?source=chemidplus&sourceid=0019388875 | |
Description | ChemIDplus is a free, web search system that provides access to the structure and nomenclature authority files used for the identification of chemical substances cited in National Library of Medicine (NLM) databases, including the TOXNET system. | |
Record name | Taurolidine | |
Source | DrugBank | |
URL | https://www.drugbank.ca/drugs/DB12473 | |
Description | The DrugBank database is a unique bioinformatics and cheminformatics resource that combines detailed drug (i.e. chemical, pharmacological and pharmaceutical) data with comprehensive drug target (i.e. sequence, structure, and pathway) information. | |
Explanation | Creative Common's Attribution-NonCommercial 4.0 International License (http://creativecommons.org/licenses/by-nc/4.0/legalcode) | |
Record name | Taurolidine | |
Source | EPA DSSTox | |
URL | https://comptox.epa.gov/dashboard/DTXSID00173001 | |
Description | DSSTox provides a high quality public chemistry resource for supporting improved predictive toxicology. | |
Record name | Taurolidine | |
Source | European Chemicals Agency (ECHA) | |
URL | https://echa.europa.eu/substance-information/-/substanceinfo/100.039.090 | |
Description | The European Chemicals Agency (ECHA) is an agency of the European Union which is the driving force among regulatory authorities in implementing the EU's groundbreaking chemicals legislation for the benefit of human health and the environment as well as for innovation and competitiveness. | |
Explanation | Use of the information, documents and data from the ECHA website is subject to the terms and conditions of this Legal Notice, and subject to other binding limitations provided for under applicable law, the information, documents and data made available on the ECHA website may be reproduced, distributed and/or used, totally or in part, for non-commercial purposes provided that ECHA is acknowledged as the source: "Source: European Chemicals Agency, http://echa.europa.eu/". Such acknowledgement must be included in each copy of the material. ECHA permits and encourages organisations and individuals to create links to the ECHA website under the following cumulative conditions: Links can only be made to webpages that provide a link to the Legal Notice page. | |
Record name | TAUROLIDINE | |
Source | FDA Global Substance Registration System (GSRS) | |
URL | https://gsrs.ncats.nih.gov/ginas/app/beta/substances/8OBZ1M4V3V | |
Description | The FDA Global Substance Registration System (GSRS) enables the efficient and accurate exchange of information on what substances are in regulated products. Instead of relying on names, which vary across regulatory domains, countries, and regions, the GSRS knowledge base makes it possible for substances to be defined by standardized, scientific descriptions. | |
Explanation | Unless otherwise noted, the contents of the FDA website (www.fda.gov), both text and graphics, are not copyrighted. They are in the public domain and may be republished, reprinted and otherwise used freely by anyone without the need to obtain permission from FDA. Credit to the U.S. Food and Drug Administration as the source is appreciated but not required. | |
Retrosynthesis Analysis
AI-Powered Synthesis Planning: Our tool employs the Template_relevance Pistachio, Template_relevance Bkms_metabolic, Template_relevance Pistachio_ringbreaker, Template_relevance Reaxys, Template_relevance Reaxys_biocatalysis model, leveraging a vast database of chemical reactions to predict feasible synthetic routes.
One-Step Synthesis Focus: Specifically designed for one-step synthesis, it provides concise and direct routes for your target compounds, streamlining the synthesis process.
Accurate Predictions: Utilizing the extensive PISTACHIO, BKMS_METABOLIC, PISTACHIO_RINGBREAKER, REAXYS, REAXYS_BIOCATALYSIS database, our tool offers high-accuracy predictions, reflecting the latest in chemical research and data.
Strategy Settings
Precursor scoring | Relevance Heuristic |
---|---|
Min. plausibility | 0.01 |
Model | Template_relevance |
Template Set | Pistachio/Bkms_metabolic/Pistachio_ringbreaker/Reaxys/Reaxys_biocatalysis |
Top-N result to add to graph | 6 |
Feasible Synthetic Routes
Disclaimer and Information on In-Vitro Research Products
Please be aware that all articles and product information presented on BenchChem are intended solely for informational purposes. The products available for purchase on BenchChem are specifically designed for in-vitro studies, which are conducted outside of living organisms. In-vitro studies, derived from the Latin term "in glass," involve experiments performed in controlled laboratory settings using cells or tissues. It is important to note that these products are not categorized as medicines or drugs, and they have not received approval from the FDA for the prevention, treatment, or cure of any medical condition, ailment, or disease. We must emphasize that any form of bodily introduction of these products into humans or animals is strictly prohibited by law. It is essential to adhere to these guidelines to ensure compliance with legal and ethical standards in research and experimentation.