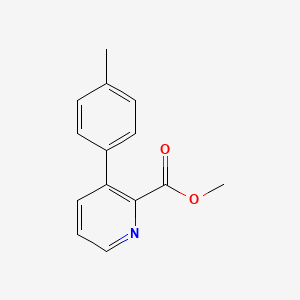
Methyl 3-(p-tolyl)picolinate
- Click on QUICK INQUIRY to receive a quote from our team of experts.
- With the quality product at a COMPETITIVE price, you can focus more on your research.
Overview
Description
Methyl 3-(p-tolyl)picolinate is an organic compound that belongs to the class of picolinates It is characterized by a picolinic acid core with a methyl ester group and a p-tolyl substituent
Preparation Methods
Synthetic Routes and Reaction Conditions: The synthesis of Methyl 3-(p-tolyl)picolinate typically involves the esterification of 3-(p-tolyl)picolinic acid with methanol in the presence of an acid catalyst. The reaction is carried out under reflux conditions to ensure complete conversion. The general reaction scheme is as follows: [ \text{3-(p-tolyl)picolinic acid} + \text{methanol} \xrightarrow{\text{acid catalyst}} \text{this compound} + \text{water} ]
Industrial Production Methods: In an industrial setting, the production of this compound can be scaled up using continuous flow reactors. This method allows for better control over reaction conditions, such as temperature and pressure, leading to higher yields and purity of the final product.
Types of Reactions:
Oxidation: this compound can undergo oxidation reactions, typically using reagents like potassium permanganate or chromium trioxide, to form corresponding carboxylic acids.
Reduction: Reduction of this compound can be achieved using reducing agents such as lithium aluminum hydride, leading to the formation of alcohol derivatives.
Substitution: The aromatic ring in this compound can undergo electrophilic substitution reactions, such as nitration or halogenation, using reagents like nitric acid or halogens.
Common Reagents and Conditions:
Oxidation: Potassium permanganate in acidic or neutral conditions.
Reduction: Lithium aluminum hydride in anhydrous ether.
Substitution: Nitric acid for nitration, halogens for halogenation.
Major Products Formed:
Oxidation: 3-(p-tolyl)picolinic acid.
Reduction: 3-(p-tolyl)picolinyl alcohol.
Substitution: Nitro or halogenated derivatives of this compound.
Scientific Research Applications
Methyl 3-(p-tolyl)picolinate has several applications in scientific research:
Chemistry: It is used as an intermediate in the synthesis of more complex organic molecules.
Biology: This compound can be used in the study of enzyme interactions and as a ligand in coordination chemistry.
Industry: Used in the production of agrochemicals and as a precursor for various functional materials.
Mechanism of Action
The mechanism of action of Methyl 3-(p-tolyl)picolinate involves its interaction with specific molecular targets, such as enzymes or receptors. The picolinic acid moiety can chelate metal ions, influencing various biochemical pathways. The p-tolyl group can enhance the compound’s lipophilicity, facilitating its interaction with lipid membranes and proteins.
Comparison with Similar Compounds
- Methyl 3-(p-methylphenyl)picolinate
- Methyl 3-(p-chlorophenyl)picolinate
- Methyl 3-(p-nitrophenyl)picolinate
Comparison: Methyl 3-(p-tolyl)picolinate is unique due to the presence of the p-tolyl group, which imparts specific electronic and steric properties. Compared to its analogs, such as Methyl 3-(p-chlorophenyl)picolinate, it exhibits different reactivity and interaction profiles, making it suitable for distinct applications in research and industry.
Properties
Molecular Formula |
C14H13NO2 |
---|---|
Molecular Weight |
227.26 g/mol |
IUPAC Name |
methyl 3-(4-methylphenyl)pyridine-2-carboxylate |
InChI |
InChI=1S/C14H13NO2/c1-10-5-7-11(8-6-10)12-4-3-9-15-13(12)14(16)17-2/h3-9H,1-2H3 |
InChI Key |
MVYZZDHMGNQOLP-UHFFFAOYSA-N |
Canonical SMILES |
CC1=CC=C(C=C1)C2=C(N=CC=C2)C(=O)OC |
Origin of Product |
United States |
Disclaimer and Information on In-Vitro Research Products
Please be aware that all articles and product information presented on BenchChem are intended solely for informational purposes. The products available for purchase on BenchChem are specifically designed for in-vitro studies, which are conducted outside of living organisms. In-vitro studies, derived from the Latin term "in glass," involve experiments performed in controlled laboratory settings using cells or tissues. It is important to note that these products are not categorized as medicines or drugs, and they have not received approval from the FDA for the prevention, treatment, or cure of any medical condition, ailment, or disease. We must emphasize that any form of bodily introduction of these products into humans or animals is strictly prohibited by law. It is essential to adhere to these guidelines to ensure compliance with legal and ethical standards in research and experimentation.