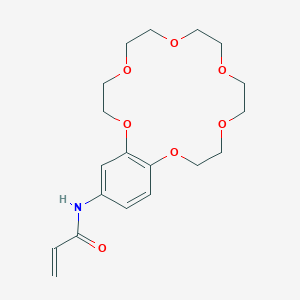
4-Acryloylamidobenzo-18-crown-6
Overview
Description
4-Acryloylamidobenzo-18-crown-6 (CAS: 68865-32-7) is a functionalized crown ether derivative with a molecular formula of C₁₉H₂₇NO₇ and a molecular weight of 381.42 g/mol . Its structure consists of an 18-membered macrocyclic ether ring (18-crown-6) fused to a benzene ring, modified with an acryloylamide group at the 4-position. This functionalization enables covalent incorporation into polymer matrices, making it a critical component in advanced material synthesis .
Preparation Methods
The synthesis of 4-Acryloylamidobenzo-18-crown-6 can be achieved through several synthetic routes. A common method involves the reaction of benzo-18-crown-6 with acryloyl chloride in the presence of a base such as triethylamine . The reaction typically proceeds under mild conditions, and the product is purified through recrystallization or chromatography . Industrial production methods may involve similar synthetic routes but on a larger scale, with optimization for yield and purity .
Chemical Reactions Analysis
4-Acryloylamidobenzo-18-crown-6 undergoes various chemical reactions, including:
Substitution Reactions: The acryloylamide group can participate in nucleophilic substitution reactions, where nucleophiles replace the acryloyl group.
Polymerization: The acryloyl group can undergo free radical polymerization, forming polymers with crown ether functionalities.
Complexation: The crown ether moiety can form stable complexes with metal ions, particularly alkali and alkaline earth metals.
Common reagents used in these reactions include bases like triethylamine, radical initiators for polymerization, and metal salts for complexation . The major products formed depend on the specific reaction conditions and reagents used.
Scientific Research Applications
Analytical Chemistry
Ion Selectivity and Detection:
4-Acryloylamidobenzo-18-crown-6 exhibits high selectivity for certain metal ions, particularly lithium and potassium. Its ability to form stable complexes with these cations makes it an excellent candidate for use in ion-selective electrodes and sensors. For instance, studies have shown that functionalizing electrodes with this compound enhances their sensitivity and selectivity towards potassium ions, facilitating real-time monitoring in various applications such as biological systems and environmental samples .
Complexation Studies:
Research indicates that this compound can form complexes with transition metal ions, which can be analyzed using spectroscopic techniques. This property is valuable for developing new analytical methods for detecting metal ions in complex matrices, such as biological fluids or industrial effluents .
Materials Science
Polymer Chemistry:
The incorporation of this compound into polymer matrices has been explored for creating functional materials with enhanced properties. For example, copolymers containing this crown ether have been synthesized to improve the ionic conductivity and mechanical strength of the resulting materials. These polymers can be utilized in applications such as solid-state batteries and electrochemical devices .
Nanocomposites:
This compound has also been investigated as a modifier in the synthesis of nanocomposites. By integrating this compound with nanoparticles (such as gold or silver), researchers have developed materials that exhibit unique optical and electronic properties, suitable for sensors and catalysis .
Environmental Monitoring
Heavy Metal Detection:
One of the significant applications of this compound is in the detection of heavy metals in wastewater and environmental samples. Its ability to selectively bind to specific metal ions allows for the development of sensitive detection methods that can be used in environmental monitoring programs. For instance, modified sensors using this compound have shown promise in detecting lead ions at low concentrations .
Phase Transfer Catalysis:
In organic synthesis, this compound can act as a phase transfer catalyst, facilitating reactions between reactants in different phases (e.g., aqueous and organic). This property is particularly useful in enhancing the solubility of otherwise insoluble salts, thus improving reaction yields and rates .
Case Study 1: Potassium Ion Detection
A study demonstrated the use of this compound functionalized electrodes for potassium ion detection in biological fluids. The electrodes showed a linear response to potassium concentrations ranging from nanomolar to micromolar levels, indicating their potential for clinical diagnostics.
Case Study 2: Heavy Metal Ion Sensors
Another research project focused on developing a sensor for lead ion detection using a composite material made from this compound and gold nanoparticles. The sensor exhibited high sensitivity and selectivity towards lead ions, making it suitable for environmental monitoring applications.
Mechanism of Action
The mechanism of action of 4-Acryloylamidobenzo-18-crown-6 primarily involves its ability to form stable complexes with metal ions. The crown ether moiety provides a cavity that can encapsulate metal ions, stabilizing them through electrostatic interactions and coordination bonds . This complexation ability is crucial for its applications in sensing, catalysis, and material science .
Comparison with Similar Compounds
Physical Properties :
Applications :
The acryloylamide group allows photopolymerization, facilitating its use in hydrogel-based sensors for heavy metal detection (e.g., Pb²⁺) via optical interference . It is also employed in ion-selective membranes to mitigate leaching issues common in plasticized polymeric sensors .
4-Acryloylamidobenzo-15-crown-5 (AAB15C5)
Structural Differences :
- Crown Size: 15-membered ring (15-crown-5) vs. 18-membered (18-crown-6).
- Functional Group: Acryloylamide at the 4-position.
Ion Selectivity :
- AAB15C5 preferentially binds Na⁺ (cavity size ~1.7 Å), while 4-Acryloylamidobenzo-18-crown-6 binds K⁺ (cavity size ~2.6 Å) due to size compatibility .
Dibenzo-18-crown-6 Derivatives (DBAP, DBMAP, DBNAP)
Structural Differences :
- Dibenzo-18-crown-6 has two benzene rings fused to the crown ether, whereas this compound has one benzene ring and an acryloylamide group.
- Derivatives like DBAP (4,4-diformyl(2-aminophenol)dibenzo-18-crown-6) feature aldimine substituents for enhanced metal coordination .
Functional Properties :
- DBAP/DBMAP/DBNAP lack polymerizable groups but exhibit tunable electron density via substituents (e.g., nitro groups in DBNAP improve heavy metal adsorption) .
- This compound’s acryloylamide enables covalent hydrogel integration, unlike dibenzo derivatives .
Parameter | This compound | Dibenzo-18-crown-6 (DBAP) |
---|---|---|
Molecular Weight (g/mol) | 381.42 | ~420 (estimated) |
Key Functional Group | Acryloylamide | Aldimine |
Application | Polymer-integrated sensors | Metal ion adsorption |
4′-Aminodibenzo-18-crown-6
Structural Differences :
- Features an amino group (-NH₂) instead of acryloylamide.
Functional Properties :
- The amino group enhances solubility in polar solvents and enables conjugation with biomolecules (e.g., proteins, DNA) .
- This compound is superior for polymerization but less reactive in biological assays.
Parameter | This compound | 4′-Aminodibenzo-18-crown-6 |
---|---|---|
Molecular Weight (g/mol) | 381.42 | 327.37 |
Key Functional Group | Acryloylamide | Amino (-NH₂) |
Application | Hydrogel sensors | Protein/DNA studies |
Benzo-18-crown-6 and Dibenzo-24-crown-8
Structural Differences :
- Benzo-18-crown-6 has one benzene ring; dibenzo-24-crown-8 has two benzene rings and a larger 24-membered ring.
Ion Selectivity :
- Benzo-18-crown-6 binds K⁺ (log K ≈ 2.1), while dibenzo-24-crown-8 targets larger ions like Cs⁺ .
- This compound offers intermediate selectivity but with polymer compatibility .
Parameter | This compound | Benzo-18-crown-6 | Dibenzo-24-crown-8 |
---|---|---|---|
Molecular Weight (g/mol) | 381.42 | 360.40 | ~500 (estimated) |
Cavity Size (Å) | 2.6 | 2.6 | 3.4 |
Key Ion Target | K⁺ | K⁺ | Cs⁺ |
Biological Activity
4-Acryloylamidobenzo-18-crown-6 (AAB18C6) is a synthetic compound belonging to the crown ether family, known for its ability to selectively bind cations and small molecules. Its unique structure, which includes an acryloylamide functional group, enhances its potential applications in biological systems, particularly in sensing and ion-selective membranes.
AAB18C6 has the molecular formula CHN O and a molecular weight of 373.43 g/mol. The presence of the crown ether moiety allows it to form complexes with various metal ions, including potassium (K), sodium (Na), and lead (Pb). This property is critical for its biological activity, particularly in sensing applications.
The biological activity of AAB18C6 can be attributed to its ability to interact with specific ions and biomolecules. The mechanism involves:
- Ion Binding : AAB18C6 selectively binds cations through its crown ether ring, facilitating the transport of ions across membranes or into cells.
- Molecular Recognition : The compound can recognize and interact with amino acids and other biomolecules, enhancing its specificity in various applications .
Biological Applications
The biological applications of AAB18C6 are diverse, including:
- Ion Selective Membranes : AAB18C6 has been utilized as an ionophore in polymeric membranes for the selective detection of cations. Research indicates that it exhibits high selectivity for K ions over Na, making it suitable for applications in biosensors .
- Lead Ion Removal : Studies have shown that microgels incorporating AAB18C6 can effectively adsorb lead(II) ions from aqueous solutions, demonstrating potential for environmental remediation .
- Sensing Applications : AAB18C6 has been employed in supramolecular sensors that leverage its binding properties for detecting specific analytes, including amino acids and metal ions. Its incorporation into membrane sensors enhances sensitivity and selectivity .
Case Study 1: Ion Selectivity in Membrane Sensors
In a study examining the performance of AAB18C6 in polymeric membrane sensors, it was found that the compound exhibited a Nernstian response to K concentrations, with a detection limit significantly lower than traditional methods. The selectivity coefficient for K over Na was reported as log K(K, Na) = -3.0, indicating strong selectivity for potassium ions .
Case Study 2: Environmental Remediation
Research into the use of AAB18C6-functionalized microgels demonstrated their effectiveness in removing lead(II) ions from contaminated water sources. The microgels showed a significant adsorption capacity for Pb, with results suggesting they could be utilized in practical applications for environmental cleanup .
Data Tables
Property | Value |
---|---|
Molecular Formula | CHN O |
Molecular Weight | 373.43 g/mol |
Ion Selectivity | K > Na |
Detection Limit (K) | log K(K, Na) = -3.0 |
Lead(II) Ion Adsorption Capacity | High |
Q & A
Basic Research Questions
Q. What are the optimal synthetic strategies for 4-acryloylamidobenzo-18-crown-6, and how can reaction yields be improved?
To optimize synthesis, response surface methodology (RSM) is recommended. Key parameters include reaction temperature (49.15°C), reaction time (72 hours), and molar ratios (e.g., Cs₂CO₃/TBC = 3.22). These conditions maximize yields (43.38%) by balancing cyclization efficiency and template effects . Pre-purification of intermediates (e.g., 4-tert-butyl catechol) and inert atmosphere usage (nitrogen) minimize side reactions.
Q. Which spectroscopic techniques are most effective for characterizing this compound?
- Nuclear Magnetic Resonance (NMR) : For structural confirmation, and NMR resolve crown ether ring protons and acryloylamido group signals.
- Infrared (IR) Spectroscopy : Identifies functional groups (e.g., C=O stretch at ~1650 cm⁻¹, crown ether C-O-C vibrations).
- X-ray Crystallography : Resolves crystal packing and molecular geometry, as demonstrated for analogous crown ethers like 3'-formyl-benzo-15-crown-5 .
Q. How does this compound selectively bind alkali metal ions, and what experimental methods validate this selectivity?
The crown ether’s 18-membered ring selectively binds K⁺ due to size compatibility (cavity diameter ~2.6–3.2 Å). Competitive binding studies using fluorometric optical fiber sensors (OFS) in serum or urine matrices confirm selectivity via fluorescence quenching upon K⁺ complexation. Reversibility and short reaction times (~seconds) make OFS ideal for real-time monitoring .
Advanced Research Questions
Q. How do binding kinetics and diffusion coefficients of Pb²⁺ in this compound hydrogels influence sensor performance?
Using square wave voltammetry , researchers determined:
- Log Kc (binding constant) : 2.75 ± 0.014 in gels vs. 3.01 ± 0.010 in solution, indicating slightly weaker binding in polymer matrices.
- Diffusion coefficients : 6.72 × 10⁻⁶ cm²/s (control gel) vs. location-dependent values (2.84–12.60 × 10⁻⁷ cm²/s) in sensor gels, attributed to crown ether heterogeneity. Optimize homogeneity via controlled radical polymerization to reduce diffusion limitations .
Q. What methodologies resolve contradictions in reported binding constants for this compound with transition metals?
Discrepancies arise from solvent polarity, ionic strength, and measurement techniques. Standardize assays using:
- Isothermal Titration Calorimetry (ITC) : Directly measures thermodynamic parameters (ΔH, ΔS).
- UV-Vis Titration : Quantifies complexation via absorbance changes (e.g., bathochromic shifts in ligand-metal complexes).
- Electrochemical Impedance Spectroscopy (EIS) : Evaluates ion transport barriers in polymer matrices .
Q. How can computational modeling enhance the design of crown ether-based sensors?
- Molecular Dynamics (MD) Simulations : Predict ligand-metal binding geometries and stability (e.g., Pb²⁺ coordination with crown ether oxygen atoms).
- Density Functional Theory (DFT) : Calculate electronic properties (e.g., HOMO-LUMO gaps) to optimize redox-active sensor components .
Q. Methodological Considerations
Q. What strategies mitigate crown ether leaching in polymer matrices during long-term sensor use?
- Covalent Immobilization : Graft this compound via acrylamide groups into hydrogel networks.
- Crosslinking Optimization : Adjust crosslinker (e.g., MBA) concentration to balance porosity and ligand retention .
Q. How do temperature and pH affect the structural stability of this compound complexes?
- Thermal Stability : Differential Scanning Calorimetry (DSC) shows decomposition >200°C. Below 153 K, molecular motions (e.g., crown ether ring rotation) freeze, stabilizing complexes .
- pH Sensitivity : Protonation of the acryloylamido group at pH < 4 disrupts metal binding. Use buffered media (pH 6–8) for optimal performance .
Properties
IUPAC Name |
N-(2,5,8,11,14,17-hexaoxabicyclo[16.4.0]docosa-1(18),19,21-trien-20-yl)prop-2-enamide | |
---|---|---|
Source | PubChem | |
URL | https://pubchem.ncbi.nlm.nih.gov | |
Description | Data deposited in or computed by PubChem | |
InChI |
InChI=1S/C19H27NO7/c1-2-19(21)20-16-3-4-17-18(15-16)27-14-12-25-10-8-23-6-5-22-7-9-24-11-13-26-17/h2-4,15H,1,5-14H2,(H,20,21) | |
Source | PubChem | |
URL | https://pubchem.ncbi.nlm.nih.gov | |
Description | Data deposited in or computed by PubChem | |
InChI Key |
UGHKKCVGQTWASH-UHFFFAOYSA-N | |
Source | PubChem | |
URL | https://pubchem.ncbi.nlm.nih.gov | |
Description | Data deposited in or computed by PubChem | |
Canonical SMILES |
C=CC(=O)NC1=CC2=C(C=C1)OCCOCCOCCOCCOCCO2 | |
Source | PubChem | |
URL | https://pubchem.ncbi.nlm.nih.gov | |
Description | Data deposited in or computed by PubChem | |
Molecular Formula |
C19H27NO7 | |
Source | PubChem | |
URL | https://pubchem.ncbi.nlm.nih.gov | |
Description | Data deposited in or computed by PubChem | |
DSSTOX Substance ID |
DTXSID50369235 | |
Record name | ST50759498 | |
Source | EPA DSSTox | |
URL | https://comptox.epa.gov/dashboard/DTXSID50369235 | |
Description | DSSTox provides a high quality public chemistry resource for supporting improved predictive toxicology. | |
Molecular Weight |
381.4 g/mol | |
Source | PubChem | |
URL | https://pubchem.ncbi.nlm.nih.gov | |
Description | Data deposited in or computed by PubChem | |
CAS No. |
68865-32-7 | |
Record name | ST50759498 | |
Source | EPA DSSTox | |
URL | https://comptox.epa.gov/dashboard/DTXSID50369235 | |
Description | DSSTox provides a high quality public chemistry resource for supporting improved predictive toxicology. | |
Retrosynthesis Analysis
AI-Powered Synthesis Planning: Our tool employs the Template_relevance Pistachio, Template_relevance Bkms_metabolic, Template_relevance Pistachio_ringbreaker, Template_relevance Reaxys, Template_relevance Reaxys_biocatalysis model, leveraging a vast database of chemical reactions to predict feasible synthetic routes.
One-Step Synthesis Focus: Specifically designed for one-step synthesis, it provides concise and direct routes for your target compounds, streamlining the synthesis process.
Accurate Predictions: Utilizing the extensive PISTACHIO, BKMS_METABOLIC, PISTACHIO_RINGBREAKER, REAXYS, REAXYS_BIOCATALYSIS database, our tool offers high-accuracy predictions, reflecting the latest in chemical research and data.
Strategy Settings
Precursor scoring | Relevance Heuristic |
---|---|
Min. plausibility | 0.01 |
Model | Template_relevance |
Template Set | Pistachio/Bkms_metabolic/Pistachio_ringbreaker/Reaxys/Reaxys_biocatalysis |
Top-N result to add to graph | 6 |
Feasible Synthetic Routes
Disclaimer and Information on In-Vitro Research Products
Please be aware that all articles and product information presented on BenchChem are intended solely for informational purposes. The products available for purchase on BenchChem are specifically designed for in-vitro studies, which are conducted outside of living organisms. In-vitro studies, derived from the Latin term "in glass," involve experiments performed in controlled laboratory settings using cells or tissues. It is important to note that these products are not categorized as medicines or drugs, and they have not received approval from the FDA for the prevention, treatment, or cure of any medical condition, ailment, or disease. We must emphasize that any form of bodily introduction of these products into humans or animals is strictly prohibited by law. It is essential to adhere to these guidelines to ensure compliance with legal and ethical standards in research and experimentation.